Introduction: Apple Production, Storage and Rots
Apples have an estimated annual farm gate value of nearly $4 billion dollars in the United States, with downstream revenues exceeding $15 billion (US Apple Association). Apples are stored for extended periods of time (up to six months at 1°C in air, and for one year maximum in controlled atmosphere) to preserve their quality and provide fruit yearlong to meet customer demands. During storage, fungal rots can cause significant amounts of decay resulting in product losses, reduced quality, and lower economic returns for producers. The three most problematic rot causing fungi in the United States are Botrytis cinerea (gray mold), Penicillium spp. (blue mold), and Colletotrichum spp. (bitter rot) (Figures 1A-C). Both gray mold and bitter rot occur in the field and during storage. However, Penicillium expansum and other Penicillium spp., are found exclusively in storage and are also economically important (Xiao and Boal, 2009). The focus of this article will be on the blue mold fungus, but the information contained here is applicable to other fungal rot pathogens as well.
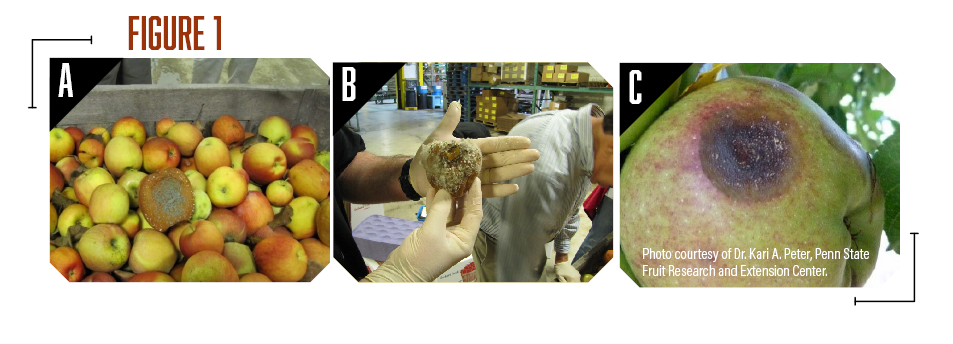
Blue Mold Biology
A survey of postharvest diseases in Washington State revealed that blue mold accounted for 28 percent of decay in storage (Kim and Xiao, 2008). Blue mold is characterized by a soft, watery rot that is light brown in color accompanied by the appearance of blue-green colored conidia on the fruit surface that develops at advanced stages of decay. P. expansum and other Penicillium spp. do not directly infect fruits, as they require wounds often caused by stem punctures and severe bruises that occur before, during, and after harvest (Figure 1A). Blue mold spreads by spores that are produced terminally in chains on the surface of whorled conidiophores (Figures 2A and B, see page 6). Stem punctures, during harvest and handling, provide places for rot to occur, but the fungus can also enter natural openings like lenticels, open calyx/sinus, and stem pull areas. Penicillium spp. also produce mycotoxins, such as patulin, citrinin, and penicillic acid, that pose potential human health risks when blue mold infected fruit are used to make juices and other processed products (Figures 3A-C). However, of the three mycotoxins, only patulin is regulated by the Food and Drug Administration (FDA) and European Food Safety Authority (EFSA) with a maximal allowable limit of 50 parts per billion (50 µg/kg-1) and 10 µg/kg-1 for babies and young infant products (European Union (EU), 2006).
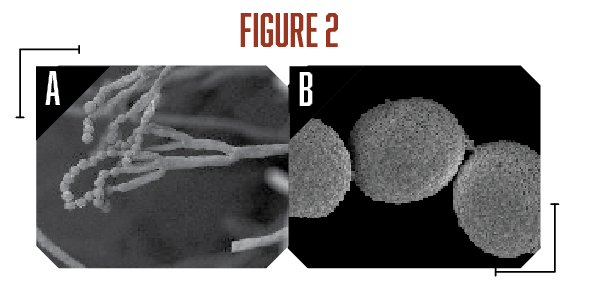
Decay Management
Postharvest fungicides
Long term storage, coupled with lack of host resistance in commercial apple fruit cultivars, provides limited options but to rely on fungicides to manage postharvest decay of apple fruit (Rosenberger, 2012). Application of postharvest fungicides depends on the stage of product handling, and is typically made by bin drenching before storage, sorting line sprays, dips in flume water, together with fruit waxing, or by thermofogging storage rooms (Figures 4A-C, see page 9). There are four postharvest fungicides (Academy, Mertect, Penbotec, Scholar) registered and being used in the United States for apple fruits to manage postharvest decay. Both Scholar (active ingredient, fludioxonil) and Penbotec (active ingredient, pyrimethanil) were labeled for postharvest use in 2004 (Xiao and Boal, 2009). Recent reports indicate reduced efficacy of these materials that have resulted in increased blue and gray mold decay in commercially stored apple fruit in Pennsylvania and Washington State (Amiri et al., 2017, Yan et al., 2014; Gaskins et al., 2015). Thiabendazole (TBZ active ingredient, Mertect®), was labeled for blue mold management in 1968 and is applied primarily as a drench. Consequently, repeated long term use of TBZ has resulted in resistant Penicillium spp. for multiple apple growing regions of the United States (e.g. New York, Pennsylvania, Maryland) and in British Columbia (Rosenberger et al., 1990; Sholberg and Haag, 1996; Jurick II personal observations). A new product (under the name Academy) was introduced in 2016, containing two single site mode of action fungicides, fludioxonil & difenoconazole, to manage postharvest decay on apples.
Sanitation
Research studies have shown that fruit bins harbor fungal spores that serve as a source of inoculum to cause rot. Implementing physical (steam) or chemical (peroxyacetic acid, quaternary amines, etc.) sanitation methods in the packinghouse to reduce inoculum levels, reduces the incidence of fruit decay. While not commonly a stand-alone tool for postharvest rot management, bin sanitation complements existing chemical controls and helps to ensure their efficacy as resistant populations are kept in check (Rosenberger, 2012; Sholberg 2004; Hansen et al., 2010). Most inoculum of Penicillium spp. comes from the orchard soil and leaf litter as this pathogen can survive very effectively as a saprophyte (Lennox et al., 2003). Therefore, bins contaminated with soil and litter introduce this fungus into the packinghouse environment which is the source for the blue mold epidemic (Figure 5A-C, see page 10). Treating bins with steam, cleaning packinghouse walls and floors with quaternary amines or peroxyacetic acids, and maintaining proper chlorine levels in sizing flumes are all important measures that can have a positive impact in managing decay (Lennox et al., 2003). Hence, studies involving quantification of blue mold spores on bin surfaces in the Pacific Northwest region concluded that bin sanitation should be a component in an integrated decay management plan (Sanderson, 2000).
Fungicide resistance monitoring
Monitoring fungicide resistance in rot fungi is critical to maintain the efficacy of single-site mode of action fungicides. This is based on developing both, conventional and molecular-based methods, to detect fungicide-resistant pathogen populations. Routine monitoring can detect shifts in baseline sensitivity of pathogens and prevent postharvest fruit losses due to fungicide resistance. Site-specific or single site mode of action chemistries have been introduced that disrupt a metabolic process, and are more prone to develop resistance. Baseline information is routinely derived from a representative pathogen population before an active ingredient is introduced to market (Russell 2004). This allows researchers to establish a Minimum Inhibitory Concentration (MIC) or discriminatory dose for a specific active ingredient generated from an “unexposed” pathogen population based on mean and the range of EC50 values. The EC50 is defined as the concentration of fungicide that reduces fungal growth by 50 percent compared to growth on non-amended media (Secor and Rivera, 2012).
Research by two collaborating groups in Washington State and Maryland have independently determined a mean EC50 for unexposed, difenoconazole-sensitive Penicillium spp. populations (Ali and Amiri, 2018; Jurick et al., 2018). Mean EC50 values for 130 P. expansum isolates from Washington State was reported to be 0.17 ppm and was 0.16 ppm for 97 Penicillium spp. isolates largely obtained from Maryland and Pennsylvania. A discriminatory dose for monitoring difenoconazole resistance should be 1 ppm or higher and up to 5 ppm to detect truly resistant isolates. Baseline data, and corresponding discriminatory doses for MIC phenotyping, have been vital in monitoring fungicide resistance in Penicillium spp. populations and identifying fungicide-resistant blue and gray mold fungi (Li and Xiao 2008; Yan et al., 2014). Our laboratory has utilized published discriminatory doses for phenotyping fungicide resistant blue and gray mold pathogens with 0.5 ppm fludioxonil, 10.0 ppm Thiabendazole and 1.0 ppm pyrimethanil in agar-based Petri plates amended with technical grade chemicals (Li and Xiao 2008).
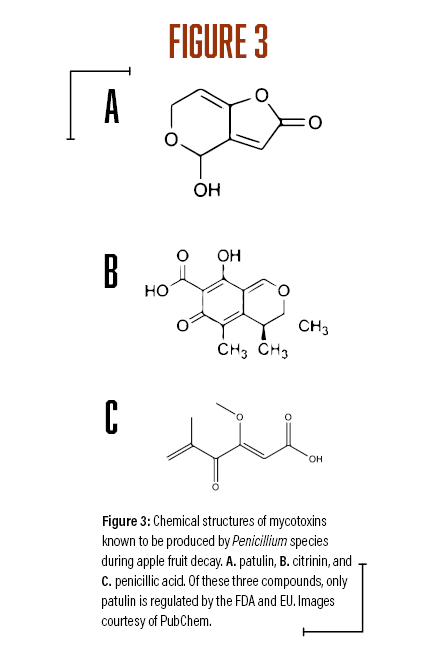
Recent Scientific Breakthroughs and Their Applications
The first genetic blueprint of the blue mold fungus was accomplished using Next Generation Sequencing Technology in the Penicillium expansum strain R19 (Yu et al., 2014). This isolate was obtained from decayed apple fruit in Pennsylvania commercial storage and shown to be highly aggressive when inoculated onto healthy apples. The P. expansum genome sequence is critical to understanding how this fungus develops resistance to various postharvest fungicides and has provided new clues about various infection strategies used by the fungus to decay apple fruit. Using sophisticated computer software analysis programs, Yu et al. determined that P. expansum R19 has 62 different secondary metabolic gene clusters and toxin biosynthetic pathways including one for patulin production. Hence, the fungus can produce a wide variety of chemicals/toxins/small molecules that may provide new uses in medicine and biotechnology as most have yet to be characterized. By sequencing and comparing different Penicillium spp. strains, genes involved in apple fruit decay, toxin production, and sexual recombination have been discovered (Julca et al., 2016; Wu et al, 2018; Yu et al., 2014). Even though the blue mold fungus has the genetic capacity to undergo sex, a definitive sexual stage for this fungus has not been observed in the laboratory or in nature. The practical impact of this discovery is that the fungus has the potential for genetic recombination, which can allow for movement of genes controlling decay, toxin secretion and/or fungicide resistance between different strains of the blue mold fungus. Hence, recombination could result in more fit strains capable of resisting multiple modes of action chemicals, and or become more aggressive resulting in increased control failures during storage.
Translating fundamental scientific information on the blue mold fungus is important and is envisioned to gain deeper insights into the genetic toolbox used by Penicillium spp. to cause decay in apple fruit. Once elucidated, the fungal tools that it uses can be exploited to develop controls that block decay from developing on apple fruit during storage. This is a similar approach to what is being done in cancer research to discover new drug targets to fight tumorigenesis, block metastatic development, and aid in early detection. Uncovering the genetic mechanisms of fungicide resistance will help tailor specific classes of new chemicals and natural products that provide durable control with lower likelihood for developing resistance. These discoveries will also enable the development of new detection tools that can be used not only by scientists, but producers as well, which would enable more timely detection of fungicide resistant isolates. Utilizing the latest molecular approaches such as CRISPR/Cas9-mediated genome editing, RNA interference, and single gene deletion strategies can be integrated to attack the fungus at multiple points that are critical for it to cause decay. These new tools for decay management, detection of fungicide resistant strains, and next generation chemistries to control postharvest rot will be a welcomed addition to the producers’ management scheme resulting in less decay, maintaining fruit quality over an extended period, and providing safer apple products devoid of mycotoxins. These breakthroughs will have wide impact and benefit our stakeholders and customers in industry, the scientific community, and the public at large.
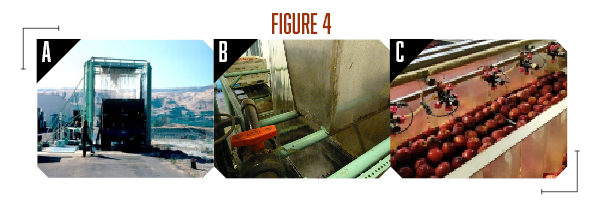
Conclusions & Summary
Postharvest decay management currently follows an integrated pest management strategy that focuses on the pathogen including sensible fungicide application, implementation of sanitation methods in the packinghouse, and periodic fungicide resistance monitoring efforts. Current knowledge concerning utilization of different postharvest chemistries based on FRAC codes (Fungicide Resistance Action Committee) suggests that rotation is critical to maintain product efficacy. However, more research must be conducted to determine the impact of chemistry type on selection of fungicide resistant rot isolates, the frequency of rotation (e.g. yearly vs. throughout the season) and impact of preharvest fungicides to predispose fungi in the field for developing resistance in storage. In the meantime, cold chain management, bin sanitation and proper fungicide use will help keep decay at acceptable levels until new controls and methods are developed, refined, and implemented. For more information on specific postharvest decay control measures, please check out online resources provided by Cornell University, Penn State, and Washington State University Extension programs via the world-wide web.
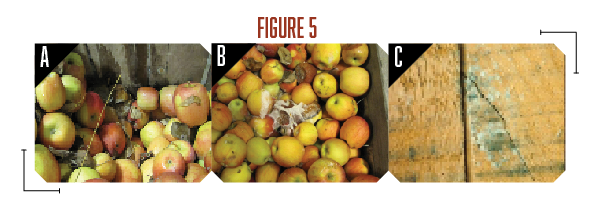
Acknowledgements
Dr. Wayne M. Jurick II is the research plant pathologist and lead scientist on the project entitled “Development of Novel Tools to Manage Fungal Plant Pathogens that Cause Postharvest Decay of Pome Fruit to Reduce Food Waste” which is funded by United States Department of Agriculture (USDA)-Agricultural Research Service (ARS) National Programs 303 Plant Diseases. Financial support was also made possible through multiple competitive grants awarded to WMJII from the State Horticultural Association of Pennsylvania.
References
Ali, E., Md. and Achour, A. 2018. Selection pressure pathways and mechanisms of resistance to the demethylation inhibitor-Difenoconazole in Penicillium expansum. Frontiers in Microbiology. doi: https://doi.org/10.3389/fmicb.2018.02472
Amiri, A., Mulvaney, K.A., and Pandit, L.K. 2017. First Report of Penicillium expansum Isolates With Low Levels of Resistance to Fludioxonil From Commercial Apple Packinghouses in Washington State. Plant Disease. 101:5.
Gaskins, V.L., Vico, I., Yu, J., and Jurick II, W.M. 2015. First report of Penicillium expansum isolates with reduced sensitivity to fludioxonil from a commercial packinghouse in Pennsylvania. Plant Dis. 99:1182.
Hansen, J., Xiao, C.L., and Kupferman, G. (2010). Bin Sanitation: an effective was to reduce codling moth and fungal decay spores. WSU publication, 1-3
Jurick II, W.M., Macarisin, O., Gaskins, V.L., Janisiewicz, W.J., Peter, K.A., and Cox, K.D. 2018. Baseline Sensitivity of Penicillium spp. to Difenoconazole. Plant Disease. 103:331-337.
Julca, I., Droby, S., Sela, N., Marcet-Houben, M., & Gabaldón, T. (2015). Contrasting genomic diversity in two closely related postharvest pathogens: Penicillium digitatum and Penicillium expansum. Genome Biology and Evolution. 8: 218– 227.
Kim, Y.K., Xiao, C.L. 2008. Distribution and incidence of Sphaeropsis rot in apple in Washington State. Plant Disease. 92:940-946.
Lennox, C.L., Spotts, R.S., and Cervantes, L.A. (2003). Populations of Botrytis cinerea and Penicillium spp. on pear fruit, and in orchards and packinghouses, and their relationship to postharvest decay. Plant Disease 87, 639-644.
Rosenberger, D.A. 1990. Blue mold. In Compendium of Apple and Pear Diseases, A.L. Jones, and H.S. Aldwinkle, eds. (Saint Paul, Minnesota, APS Press), pp. 54-55.
Rosenberger, D.A. 2012. Sanitize apple storage rooms to minimize postharvest decays. Scaffolds Fruit J 21: 4-5.
Russell, P.E. 2004. Sensitivity baselines in fungicide resistance research and management. FRAC monograph. 3:1-54.
Sanderson, P.G. 2000. Management of decay around the world and at home. 16th annual Postharvest Conference, Yakima, Washington. Pages 1-8. http://postharvest .tfrec.wsu.edu/pgDisplay.php?article=PC2000Z.
Secor, G. and Rivera, V. 2012. Fungicide resistance assays for fungal plant pathogens. In: Plant Fungal Pathogens Methods and Protocols (M.D. Bolton and B.P.H.J. Thomma, eds). New York: Springer, pp. 385-392.
Sholberg, P.L., and Haag, P.D. 1996. Incidence of postharvest pathogens of stored apples in British Columbia. Canadian Journal of Plant Pathology. 18: 81-85.
Sholberg, P.L. 2004. Bin and storage room sanitation. Washington Tree Fruit Postharvest Conference.
Wu, G., Jurick II, W.M., Lichtner, F.J., Peng, H., Yin, G., Gaskins, V.L., Yin, y., Hua, S., Peter, K.A., Bennett, J.W. 2018. Whole-genome comparisons of Penicillium spp. reveals secondary metabolic gene clusters and candidate genes associated with fungal aggressiveness during apple fruit decay. PeerJ. 1-17. 7:e6170. doi.org/10.7717/peerj.6170
Xiao, C.L., and Boal, R.J. 2009. Residual activity of fludioxonil and pyrimethanil against Penicillium expansum on apple fruit. Plant Disease 93:1003-1008.
Yan, H., Gaskins, V.L., Vico, I., Luo, Y., and Jurick II, W.M. 2014. First Report of Penicillium expansum isolates resistant to pyrimethanil from stored apple fruit in Pennsylvania. Plant Dis. 98:7.
Yan, H., Gaskins, V.L., Lou, Y., Kim, Y.K., and Jurick II, W.M. First Report of Pyrimethanil Resistance in Botrytis cinerea from Stored Apples in Pennsylvania. Plant Dis. 98:7. 2014.
Yu, J., Jurick II, W.M., Cao, H.Y., Yin, Y., Gaskins, V.L., Losada, L., Zafar, N., Kim, M., Bennett, J.W., and Nierman, W. (2014). Draft genome sequence of Penicillium expansum R19, which causes postharvest decay of apple fruit. Genome Announcements 2, e00635-00614