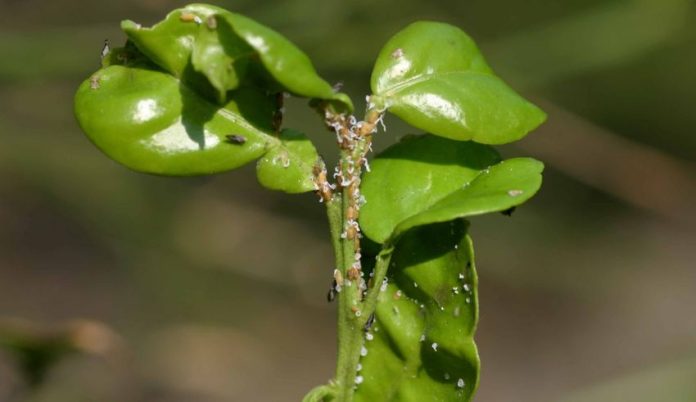
Citrus Huanglongbing (HLB, citrus greening disease) is caused by the vector-transmitted phloem-limited bacterium Candidatus Liberibacter asiaticus (CLas). It is the most destructive disease which infects all commercial citrus varieties and threatens citrus industries worldwide (Bove 2006; Graham et al. 2020). Current management strategies include insecticide application to control the transmission vector Asian citrus psyllids (ACP) and antibiotics treatment to inhibit CLas (Barnett et al. 2019), but neither could control HLB effectively. Since the first report of HLB in Florida in 2005, citrus acreage and production in Florida decreased by 38% and 74%, respectively (Graham et al. 2020; Stokstad 2012). The disease has spread to citrus-producing states, including Texas and California. In severely affected areas, such as Florida, effective therapy is demanded because disease eradication is impractical. In recently impacted areas, such as California, preventing new infections is most urgent. Hence, innovative therapeutic and preventive strategies to combat HLB are urgently needed to ensure the survival of the citrus industry.
One of the most effective and eco-friendly strategies for disease management is to utilize plant innate immunity-related genes from disease-resistant or tolerant varieties for plant protection. Upon pathogen infection, plant defense response genes undergo expression reprogramming to trigger plant innate immunity. Plant endogenous small RNAs play a pivotal role in this regulatory process (Huang et al. 2019; Zhao et al. 2013b), including phytohormone- and chemical-induced systemic acquired resistance or defense priming, which can promote robust host immune responses upon subsequent pathogen challenges (Brigitte et al. 2017; Zheng Qing and Xinnian 2013).
Our Approaches
HLB tolerance has been observed in some hybrids (e.g., US-942 and Sydney hybrid 72 (Albrecht and Bowman 2011, 2012a)) or citrus relatives (e.g., Microcitrus australiasica, Eremocitrus glauca and Poncirus trifoliata) (Ramadugu et al. 2016). By comparative analysis of small RNA profiles and the target gene expression between HLB-sensitive cultivars and HLB-tolerant citrus hybrids and relatives (Albrecht and Bowman 2011, 2012a), we identified a list of candidate natural defense genes potentially responsible for HLB tolerance (Huang et al. 2020). One of the candidate regulators is a novel anti-microbial peptide (AMP), which we named “stable antimicrobial peptide” (SAMP). Here, we demonstrate that SAMP not only has antimicrobial activity but also has priming activity and can induce citrus systemic defense responses. This dual-functional SAMP can reduce CLas titer, suppress disease symptoms in HLB-positive trees and activate plant systemic defense responses against new infection.
Results
Through the comparative expression analysis of small RNAs and the target genes between HLB-sensitive cultivars and HLB-tolerant citrus US-942 (Poncirus trifoliata x Citrus reticulata) and microcitrus Sydney hybrid 72 (Microcitrus virgate from M. australis × M. australasica) (Huang et al. 2020), we identified a list of candidate plant immune regulators that are potentially contributable to HLB-tolerance. One candidate regulator is a 67-amino acid (aa) peptide, SAMP, that was predicted with antimicrobial activity (Park et al. 2007). SAMP has significantly higher expression levels in both HLB-tolerant hybrids US-942 and Syd 72 than the HLB-susceptible control trees. We further cloned SAMP genes from HLB-tolerant citrus relatives. We found that SAMP transcripts are closely related and have a significantly higher expression level in HLB-tolerant varieties. We further detected the 6.7kD SAMP in the phloem-rich tissue; bark peels of HLB-tolerant Ma and Pt but not in the susceptible Cs. These results support that the SAMPs are likely associated with the HLB-tolerance trait. According to our functional analysis of SAMP, we list the advantages of using SAMP to manage citrus HLB as the following:
SAMP has bactericidal activity and is heat stable.
We screened SAMPs from several citrus relatives using a C. Liberibacter solanacearum (CLso)/potato psyllid/Nicotiana benthamiana interaction system to mimic the natural transmission and infection circuit of the HLB complex. We found that the SAMP from Ma Australian finger lime (MaSAMP) had the strongest effect on suppressing CLso disease and inhibiting bacterial growth in plants. To directly determine the bactericidal activity of MaSAMP on Liberbacter spp, we developed a viability/cytotoxicity assay of Lcr, a close culturable relative of the CLas and CLso (Fagen et al. 2014; Leonard et al. 2012; Merfa et al. 2019). Using this assay, we found that MaSAMP can rapidly kill the bacterial cells within five hours, which is more efficient than the bactericidal antibiotic, Streptomycin. While the heat sensitivity of antibiotics is a major drawback for controlling CLas in citrus fields, we found that SAMPs are surprisingly heat stable. A prolonged exposure to extreme temperatures of 60 degrees C for 20 hours had minimal effect on MaSAMP, which still retained most of its bactericidal activity, whereas Streptomycin lost its antibacterial activity following the same temperature incubation. Thus, SAMP is a heat-stable, plant-derived antimicrobial peptide that can directly kill Lcr and suppress CLso in plants.
SAMP suppresses CLas in HLB-positive citrus trees.
To determine whether MaSAMP can also suppress CLas in citrus trees, we used the pneumatic trunk injection method to deliver the MaSAMP solution into the HLB-positive trees. We first tested with eight CLas-positive Citrus macrophylla with similar bacterial titer and disease symptoms for the treatment. At eight weeks following two doses injected, separated by two months of MaSAMP injection, the disease symptoms and the bacterial titer in all six treated trees were drastically reduced compared to the mock-treated (buffer only) plants and one tree with no CLas detected. We further tested with HLB-positive ‘Madam Vinous’ sweet oranges and Lisbon Lemon trees; those appeared to have declining symptoms and similar CLas titer. After MaSAMP treatments, the trees had developed symptomless new flushes, while mock trees exhibited symptomatic flushes (Fig. 1). The CLas titer was reduced in MaSAMP-treated trees, while it increased in the mock-treated trees. Taken together, these results demonstrate across three trials that SAMP injection can suppress CLas titer in three different HLB-susceptible citrus varieties and can cause trees in declining health to recover.
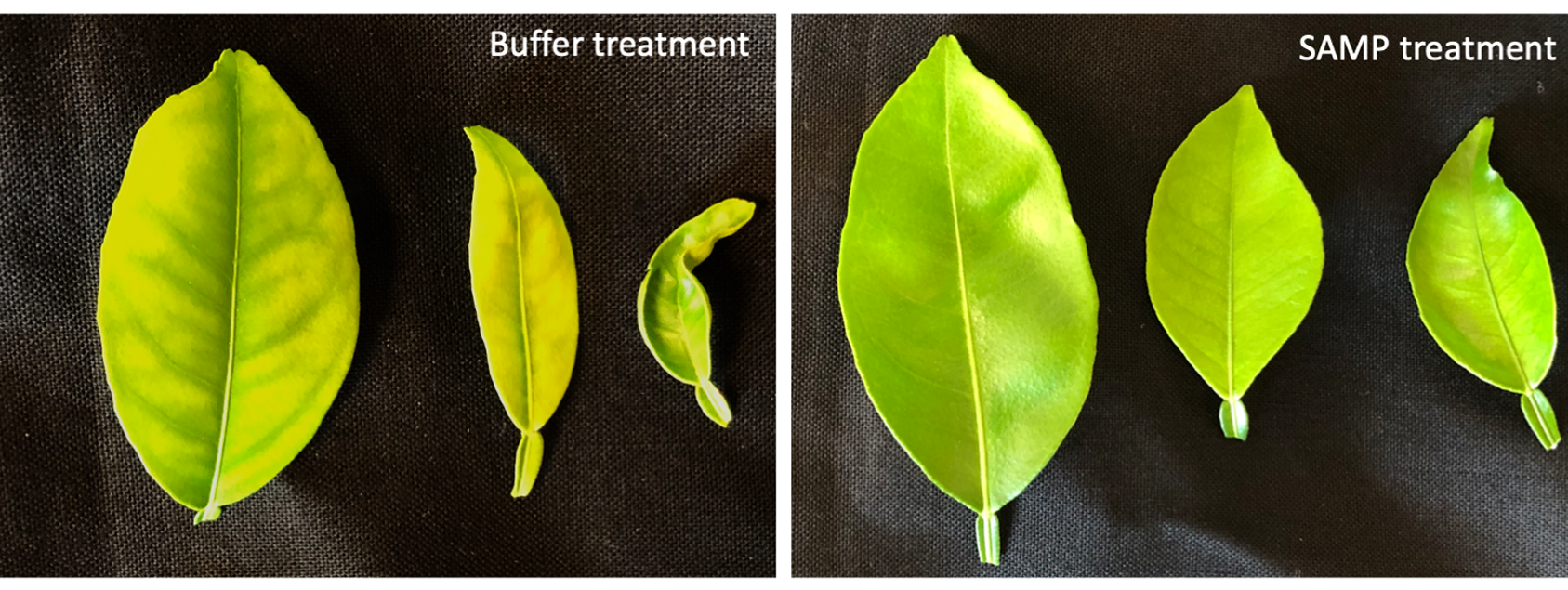
SAMP treatment safeguards healthy citrus trees from CLas infection.
Protecting healthy trees from CLas infection is critical for managing HLB. The establishment of defense priming in plants can promote faster and/or stronger host immune responses upon pathogen challenges (Brigitte et al. 2017; Zheng Qing and Xinnian 2013). To determine whether MaSAMP has priming activity, we applied it by foliar spray to citrus plants. We found that MaSAMP applications triggered prolonged induction of defense response genes. Thus, SAMP can potentially “vaccinate” uninfected citrus trees and induce defense responses to combat against HLB. To test the protection ability of SAMP on citrus trees, we applied the MaSAMP solution or buffer as mock treatment by foliar spray onto young, healthy ‘Madam Vinous’ sweet orange trees. Five days after treatment, the trees were exposed to ACP carrying CLas under the “no choice feeding” condition for 21 days. We treated trees with MaSAMP solution by foliar spray every two months subsequently. The result indicates that MaSAMP-treated trees have a lower infection rate.
SAMP disrupts the outer membrane and causes cell lysis of the bacterial cell.
To understand the mechanism of MaSAMP bactericidal activity, morphological changes of Lcr post-MaSAMP treatment were observed using transmission electron microscopy. Application of 10 μM MaSAMP to Lcr caused cytosol leakage and the release of small extracellular vesicles after 30 minutes of incubation. The Lcr cells were lysed within two hours of incubation. We isolated the membrane fraction from the MaSAMP treated Lcr and detected the enrichment of MaSAMP in the outer membrane fraction compared with the inner membrane fraction. Thus, MaSAMP likely disrupts mainly the outer membrane of Lcr and breaks the bacterial cells, which leads to cell lysis.
SAMP has Low toxicity.
Because SAMP is internalized by citrus, it is important to test its phytotoxicity. We injected different concentrations of MaSAMP solution directly into citrus leaves and found that MaSAMP has little phytotoxicity. Furthermore, we found that MaSAMP can be detected in fruit tissue of both Australian finger lime and trifoliate orange by Western blot analysis and is very sensitive to human endopeptidase Pepsin, a major gastric enzyme produced by stomach chief cells. Thus, MaSAMP in Australian finger lime has already been consumed by humans for hundreds of years and can be easily digested (Figure 2). These results suggest a low possibility of toxicity of SAMP on citrus and humans, although additional safety assessment tests are necessary for regulatory approval.
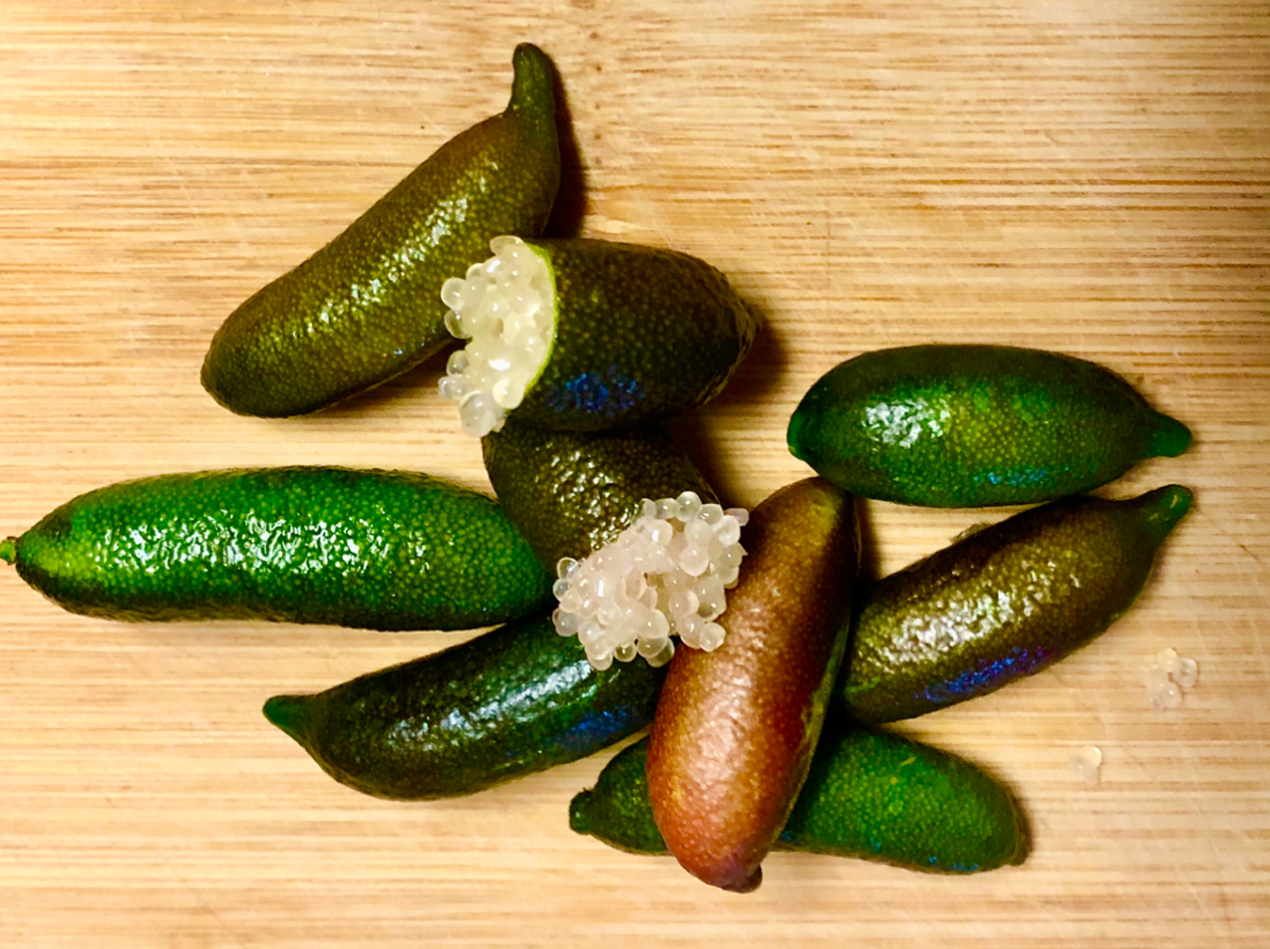
Conclusion
Current methods for HLB management include insecticidal control of the vector (Stansly et al. 2014), antibacterial treatments (Blaustein et al. 2018; Gottwald 2010; Hu et al. 2018; Zhang et al. 2014) and nutrient supplements (Rouse 2013; Zhao et al. 2013a). The overuse of insecticides and antibiotics is known to pose threats to human and animal health and select for resistance in the target insect population (Tiwari et al. 2011). Further, current bactericidal or bacteriostatic treatments mostly involve sprays of antibiotics, such as streptomycin and oxytetracycline, which are likely to select for antibiotic-resistant bacteria strains and disrupt the citrus microbiome and ecosystem and may further affect the effectiveness of these antibiotics for medical antibacterial treatment in humans and animals.
On the contrary, SAMPs have a distinct mode of action and tend to interact with the bacterial cell membrane through nonspecific mechanisms, making the emergence of resistant bacteria less likely (Jochumsen et al. 2016; Rodriguez-Rojas et al. 2014). Moreover, SAMP kills bacteria faster than antibiotics, reducing bacterial generations and further lowering the possibility of evolved resistance (Fantner et al. 2010). Most importantly, the heat stability of SAMP can provide a prolonged and durable effect in the field compared to heat-sensitive antibiotics. SAMP not only kills bacteria cells but can also prime plant immune responses to prevent/reduce infection. In our greenhouse trials, SAMP has been shown to treat HLB-positive trees and inhibit the emergence of new HLB-infection in healthy trees. Field trials, which can take several years, are currently being initiated in Florida to confirm the efficacy of SAMP in controlling HLB. Field trials also include testing multiple peptide application methods for citrus growers to prevent and treat HLB.
Contact Hailing Jin at hailingj@ucr.edu for more information.
References:
Albrecht, U., and Bowman, K.D. (2011). Tolerance of the trifoliate citrus hybrid US-897 (Citrus reticulata x Poncirus trifoliata) to huanglongbing. HortScience 46, 16-22.
Albrecht, U., and Bowman, K.D. (2012a). Tolerance of trifoliate citrus hybrids to Candidatus liberibacter asiaticus. . Sc Horticulturae 147, 71-80.
Barnett, M.J., Solow-Cordero, D.E., and Long, S.R. (2019). A high-throughput system to identify inhibitors of Candidatus Liberibacter asiaticus transcription regulators. Proceedings of the National Academy of Sciences of the United States of America 116, 18009-18014.
Blaustein, R.A., Lorca, G.L., and Teplitski, M. (2018). Challenges for Managing Candidatus Liberibacter spp. (Huanglongbing Disease Pathogen): Current Control Measures and Future Directions. Phytopathology 108, 424-435.
Bove, J.M. (2006). Huanglongbing: a destructive, newly-emerging, century-old disease of citrus. . J Plant Pathol 88, 7-37.
Brigitte, M.-M., Ivan, B., Estrella, L., and Victor, F. (2017). Defense Priming: An Adaptive Part of Induced Resistance. Annual review of plant biology 68, 485-512.
Fagen, J.R., Leonard, M.T., Coyle, J.F., McCullough, C.M., Davis-Richardson, A.G., Davis, M.J., and Triplett, E.W. (2014). Liberibacter crescens gen. nov., sp. nov., the first cultured member of the genus Liberibacter. International journal of systematic and evolutionary microbiology 64, 2461-2466.
Fantner, G.E., Barbero, R.J., Gray, D.S., and Belcher, A.M. (2010). Kinetics of antimicrobial peptide activity measured on individual bacterial cells using high-speed atomic force microscopy. Nature nanotechnology 5, 280-285.
Gottwald, T.R. (2010). Current epidemiological understanding of citrus Huanglongbing. Annu Rev Phytopathol 48, 119-139.
Graham, J., Gottwald, T., and Setamou, M. (2020). Status of Huanglongbing (HLB) outbreaks in Florida, California and Texas. Trop Plant Pathol.
Hu, J., Jiang, J., and Wang, N. (2018). Control of Citrus Huanglongbing via Trunk Injection of Plant Defense Activators and Antibiotics. Phytopathology 108, 186-195.
Huang, C., Niu, D., Kund, G., Jones, M., Albrecht, U., Nguyen, L., Bui, C., Ramadugu, C., Bowman, K., Trumble, J., et al. (2020). Identification of citrus defense regulators against citrus Huanglongbing disease and establishment of an innovative rapid functional screening system. Plant Biotechnology Journal.
Huang, C.Y., Wang, H., Hu, P., Hamby, R., and Jin, H. (2019). Small RNAs – Big Players in Plant-Microbe Interactions. Cell host & microbe 26, 173-182.
Jochumsen, N., Marvig, R.L., Damkiaer, S., Jensen, R.L., Paulander, W., Molin, S., Jelsbak, L., and Folkesson, A. (2016). The evolution of antimicrobial peptide resistance in Pseudomonas aeruginosa is shaped by strong epistatic interactions. Nature communications 7, 13002.
Leonard, M.T., Fagen, J.R., Davis-Richardson, A.G., Davis, M.J., and Triplett, E.W. (2012). Complete genome sequence of Liberibacter crescens BT-1. Standards in genomic sciences 7, 271-283.
Merfa, M.V., Perez-Lopez, E., Naranjo, E., Jain, M., Gabriel, D.W., and De La Fuente, L. (2019). Progress and Obstacles in Culturing ‘Candidatus Liberibacter asiaticus’, the Bacterium Associated with Huanglongbing. Phytopathology 109, 1092-1101.
Park, S.C., Lee, J.R., Shin, S.O., Park, Y., Lee, S.Y., and Hahm, K.S. (2007). Characterization of a heat-stable protein with antimicrobial activity from Arabidopsis thaliana. Biochemical and biophysical research communications 362, 562-567.
Ramadugu, C., Keremane, M.L., Halbert, S.E., Duan, Y.P., Roose, M.L., Stover, E., and Lee, R.F. (2016). Long-Term Field Evaluation Reveals Huanglongbing Resistance in Citrus Relatives. Plant Dis 100, 1858-1869.
Rodriguez-Rojas, A., Makarova, O., and Rolff, J. (2014). Antimicrobials, stress and mutagenesis. PLoS pathogens 10, e1004445.
Rouse, B.B. (2013). Rehabilitation of HLB Infected Citrus Trees using Severe Pruning and Nutritional Sprays. Proc Fla State Hort Soc 126, 51-54.
Stansly, P.A., Arevalo, H.A., Qureshi, J.A., Jones, M.M., Hendricks, K., Roberts, P.D., and Roka, F.M. (2014). Vector control and foliar nutrition to maintain economic sustainability of bearing citrus in Florida groves affected by huanglongbing. Pest Manag Sci 70, 415-426.
Stokstad, E. (2012). Agriculture. Dread citrus disease turns up in California, Texas. Science 336, 283-284.
Tiwari, S., Mann, R.S., Rogers, M.E., and Stelinski, L.L. (2011). Insecticide resistance in field populations of Asian citrus psyllid in Florida. Pest management science 67, 1258-1268.
Zhang, M., Guo, Y., Powell, C.A., Doud, M.S., Yang, C., and Duan, Y. (2014). Effective antibiotics against ‘Candidatus Liberibacter asiaticus’ in HLB-affected citrus plants identified via the graft-based evaluation. PloS one 9, e111032.
Zhao, H., Sun, R., Albrecht, U., Padmanabhan, C., Wang, A., Coffey, M.D., Girke, T., Wang, Z., Close, T.J., Roose, M., et al. (2013a). Small RNA profiling reveals phosphorus deficiency as a contributing factor in symptom expression for citrus huanglongbing disease. Mol Plant 6, 301-310.
Zhao, H., Sun, R., Albrecht, U., Padmanabhan, C., Wang, A., Coffey, M.D., Girke, T., Wang, Z., Close, T.J., Roose, M., et al. (2013b). Small RNA Profiling Reveals Phosphorus Deficiency as a Contributing Factor in Symptom Expression for Citrus Huanglongbing Disease. Molecular Plant 6, 301-310.
Zheng Qing, F., and Xinnian, D. (2013). Systemic Acquired Resistance: Turning Local Infection into Global Defense. Annual review of plant biology 64, 839-863.