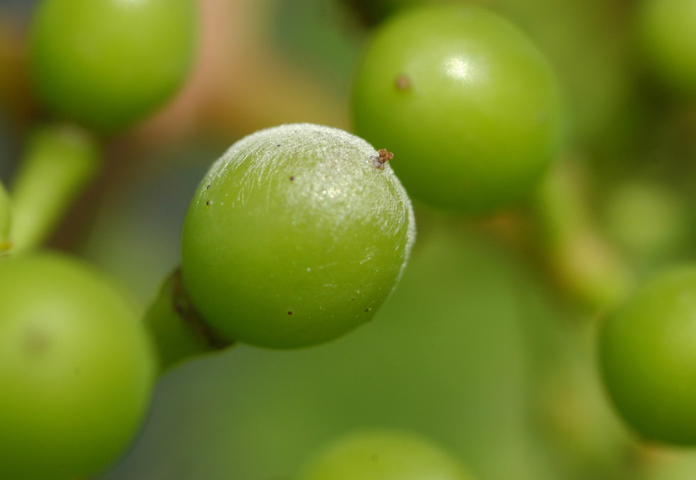
Global winegrape production is largely based upon the production of the European winegrape Vitis vinifera, a host species comprised of cultivars that are all highly susceptible to infection by the grape powdery mildew pathogen Erisyphe necator as well as several other fungal and oomycete pathogens. Irrespective of the center of origin of Vitis vinifera or the major pathogen groups, the global ubiquity of both the host and various pathogens is now a fact faced by grape and wine producers everywhere.
In particular, fungicidal suppression of grapevine powdery mildew is problematic. Resistance to many FRAC classes, including sterol demethylation inhibitors (DMI), strobilurins, benzimidazoles and succinate dehydrogenase inhibitor (SDHI) fungicides is sufficiently widespread that the forgoing classes are no longer effective in some viticultural regions. Organic production systems are also threatened. There are very few practical organic options for controlling powdery mildews. Many organic options entail undesirable non-target effects or are marginally effective. Additionally, many viticultural regions are located in Mediterranean climates with little rainfall during the crop production season. All of the foregoing creates the present situation: grapevine powdery mildew predominates as the principal threat to healthy fruit and foliage worldwide.
UV Light to Suppress Pathogens
Nearly all of the biomass of powdery mildews is wholly external to the host (Figure 1). They live in a world bathed in sunlight throughout the disease process. With the exception of the walls of their overwintering structures (chasmothecia), they possess none of the pigmentation that would offer protection from biocidal wavelengths of the solar spectrum (wavelengths of UVB between 280 and 290 nm.) Powdery mildews are favored by shade and repressed to some degree by direct sunlight exposure. They persist in the above niche due in part to their ability to repair UV-inflicted damage to their DNA through a robust photolyase mechanism driven by blue light and UVA.
In 1990, we began work that led to the use of germicidal UVC lamps to suppress E. necator. The treatments were effective, but UVC also damaged the vines, and the technology was never widely adopted (Figure 2). It took 20 years before a critical breakthrough by a Ph.D. student in Norway (Aruppillai Suthparan) fundamentally changed how we could use UV light against plant pathogens. He found that if UV light was applied during night hours, we could use much lower doses than were required during daylight. That breakthrough largely resolved the issue of plant damage at the high UV doses required for daytime applications. Today, UV technology for plant disease suppression is being investigated by several working groups. Most exploit the link between darkness and the inability to withstand exposure to UV. When damage to pathogen DNA during darkness is not repaired within four hours, it is usually lethal.
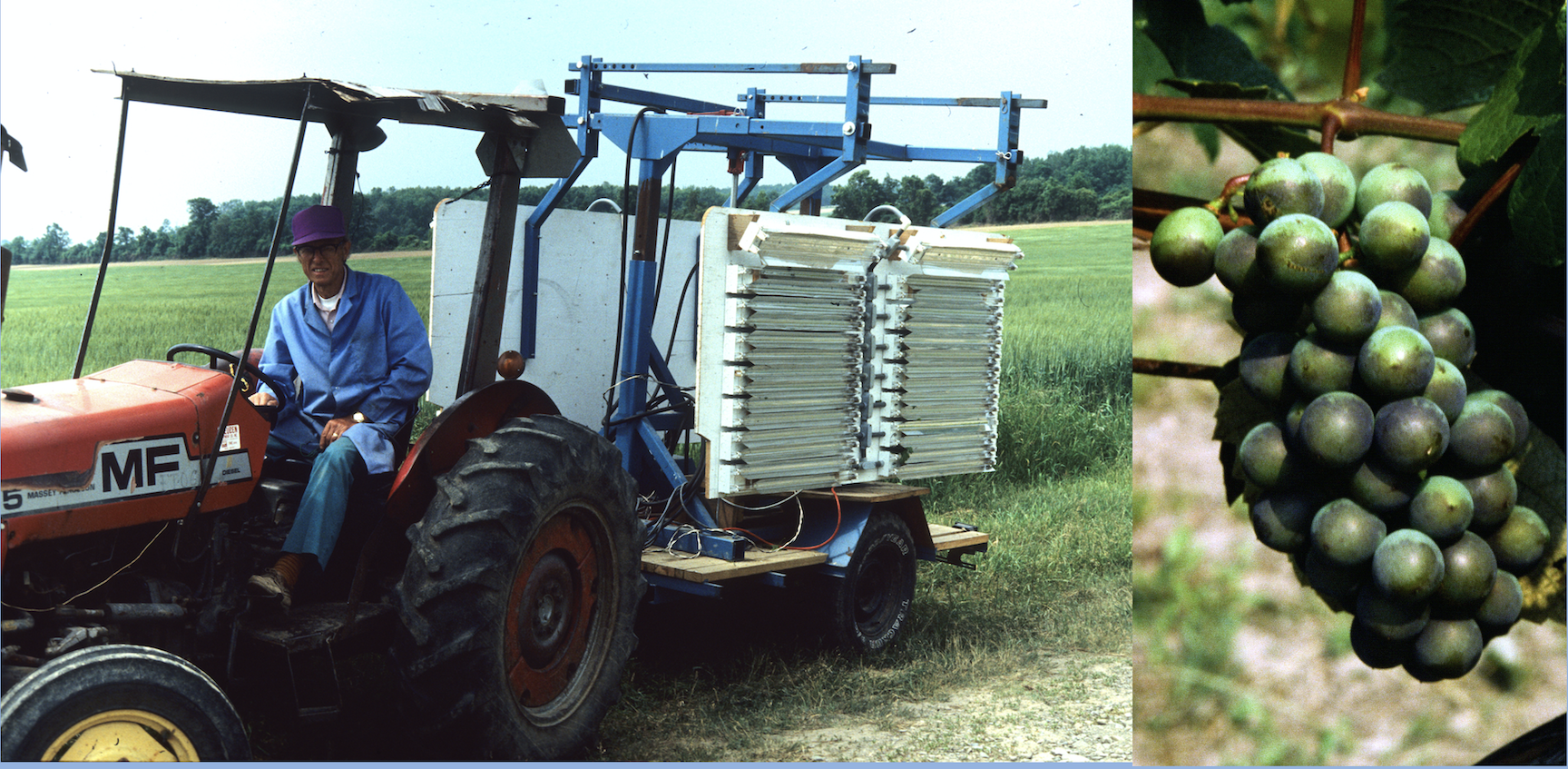
The UV spectrum used in such studies has ranged from a UVB waveband between 280 to 290 nm into the UVC range produced by low pressure discharge lamps yielding a peak output near 254 nm. Reduction of the severity of several powdery mildews has been attributed to direct damage to the pathogen by UV exposure. UVC has been reported to be directly inhibitory to Botrytis cinerea on strawberry (Janisiewicz et al. 2016). In contrast, pathogens other than powdery mildews have been suppressed by exposure of their hosts to UV prior to inoculation, possibly due to enhancement of host resistance.
The adaptation of nighttime UV treatments to commercial field plantings has necessitated the development of UV arrays powerful enough to apply effective doses at speeds that allow the equipment to complete treatments during the available night interval, often in late spring and early summer during some of the shortest nights of the year. Remember: we need about four hours of darkness after UV exposure in order to achieve the maximum suppression. A tractor-drawn UVC apparatus described in a report by Onofre et al (2019) was developed to suppress strawberry powdery mildew. This apparatus contained two hemicylindrical arrays of UVC lamps and was the basis of a later array design fitted to an autonomous robotic carriage produced by Saga Robotics, LLC. UVC treatments applied once or twice weekly at doses ranging from 70 to 200 J/m2 effectively suppressed strawberry powdery mildew (Podosphaera aphanis) to a degree that equaled or exceeded that of some of the best available fungicides.
The potential for nighttime UV treatments to eliminate the threat posed by E. necator could greatly reduce the need for fungicide applications. In regions with higher rainfall and multiple fungal pathogens, the potential for nighttime UV treatments to remove the threat of powdery mildew would improve options for the remaining members of the pathogen and pest complex, such as downy mildew (Plasmopara viticola), bunch rot (Botrytis cinerea) and various arthropod pests.
For all of the foregoing reasons, our objectives in the present study were to 1) Determine the potential of nighttime UV applications to suppress grapevine powdery mildew; 2) Determine if UVC at disease-suppressive doses and frequency of application has any deleterious effects on vine growth, yield or crop quality; and 3) Determine if nighttime UV applications targeting powdery mildew have effects on other selected pests or diseases of grapevine.
In summary, the mechanism underlying the success of nighttime UV applications is related to how pathogens deal with naturally-occurring ultraviolet light from the sun. Shorter-wave UVB and UVB both damage DNA in all living organisms. Exposure to UV causes thymine base pairs in the DNA to bind together, changing the genetic code to genetic gobbledygook. Pathogens sense visible light, but they also possess evolved systems that can repair the foregoing damage to their DNA caused by incoming UV. We now know that those biochemical and genetic repair systems are recharged by blue light and UV-A, and are reduced by red light and darkness. This photolyase-based repair mechanism effectively “unglues” the thymine base pairs as fast as they are created by UV, but the repair mechanism does not operate at night.
Lamps producing UV light have been commonly available for over 75 years. Those that produce an effective wavelength and are powerful enough to be practically used against powdery mildews produce either UVC (100 to 280 nm) or UVB (280 to 315 nm). Both UVC and UVB affect DNA in the same way by the aforementioned creation of thymine dimmers. UVB poses less potential to harm plants, and may therefore be preferred for static and permanent installations in greenhouses. However, with precise dosing, UVC can be used safely on even UV-sensitive crops.
Low-pressure discharge lamps are the most common available technology. Low-pressure discharge UVC lamps are generally clear quartz-glass tubes containing a small amount of mercury vapor. Passing an electric arc through this vapor results in the efficient production of a narrow waveband centered on 254 nm, which is excellent for germicidal applications. UVB low-pressure discharge lamps are similar, but incorporate a fluorophore powder coating on the inside of the tube. When this is struck by the internally produced UVC, the fluorophore absorbs the UVC and emits the longer wavelength UVB. This process is also relatively inefficient, and nearly 95% of the usable germicidal energy is lost in the conversion from UVC to UVB. So, low-pressure discharge UVC lamps can produce much more usable power than comparably sized UVB lamps. While UV LEDs are available, they are presently far too expensive and underpowered to be useful for treating crops.
Results Adapted to Grapevine
Field trials for suppression of strawberry powdery mildew were initiated in Florida in 2017. Weekly applications of UVC provided suppression of foliar powdery mildew across the duration of the experiment that was substantially better than that provided by the best fungicide treatment in the trial, which was a combination of two materials sold under the trade names Quintec and Torino. We also confirmed in parallel measurements that the UV treatments did not reduce plant size or the yield of harvested berries. Continued trials on field plantings of strawberries duplicated the efficacy of the 2017 trials.
In our initial trials, we used a tractor-drawn array (Figure 3). Additional trials adapted modified designs of the original tractor-drawn array to an autonomous robotic device (Figure 4) manufactured by SAGA Robotics, a Norwegian company collaborating with our research group in developing this technology for multiple crops. The use of a robotic carriage provides additional flexibility in nighttime applications. At temperate latitudes, the duration of night near the summer solstice can be less than eight hours, leaving only about four hours during which the UV treatments could be applied with optimal effect. In situations where employing nighttime labor to make applications split over several relatively short night intervals would be problematic, an autonomous robotic device offers a practical alternative.
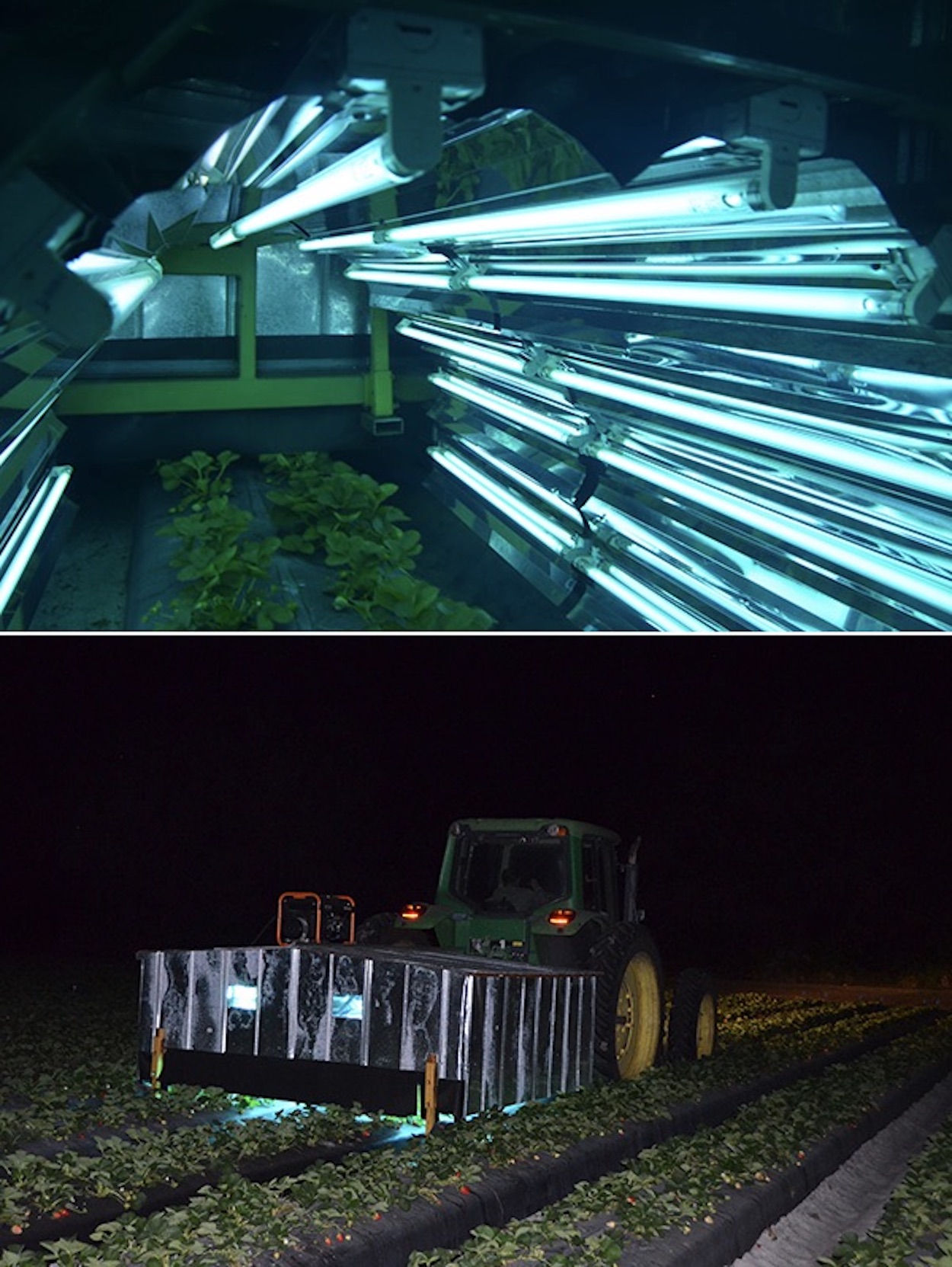
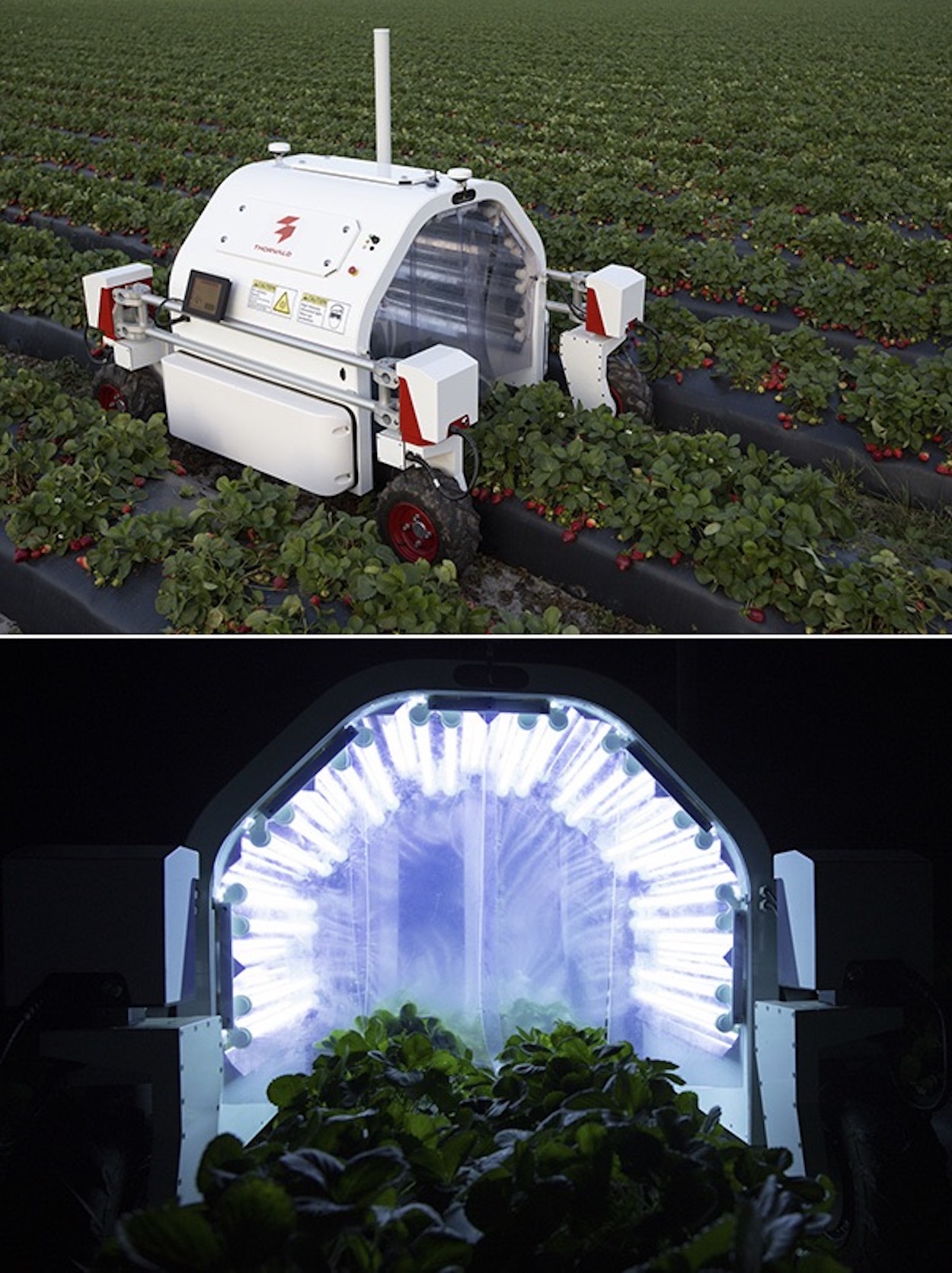
In 2019, we came full circle and were ready to resume UV treatments on grapevine. As in our work on strawberry, we began by using a UV array and tractor-drawn carriage. UV Treatments were applied once per week at 100 or 200 J/m2 to Chardonnay vines that received no other fungicide treatments. Laboratory experiments had indicated that the UV doses used would stop 80% to nearly 100% of the conidia of E. necator from germinating. The incidence and severity of powdery mildew was assessed on leaves and fruit of UV treated vines, vines treated with an effective conventional fungicide and completely untreated vines. 2019 was a moderately severe year for powdery mildew.
Both the 100 J/m2 and 200 J/m2 UVC treatments significantly but equivalently reduced the severity of powdery mildew on berries compared to the untreated vines, albeit not to the degree provided by the standard fungicide treatments (Figure 5). What surprised us was that both the 100 J/m2 and 200 J/m2 UV treatments also suppressed foliar downy mildew (Plasmopara viticola), and did so better than the fungicide standard (Figure 6). Laboratory studies indicated that the suppression of the downy mildew pathogen was due to a pre-inoculation increase in host resistance. This was distinct from the impact of UV on powdery mildew, which was primarily a direct effect of UV on the pathogen itself. However, in our 2020 trials, weather conditions were especially conducive to downy mildew, and the level of suppression of downy mildew from UV was only around 50%. That’s helpful, but it is nowhere near acceptable commerical control. So, we obviously have more work to do in this area.
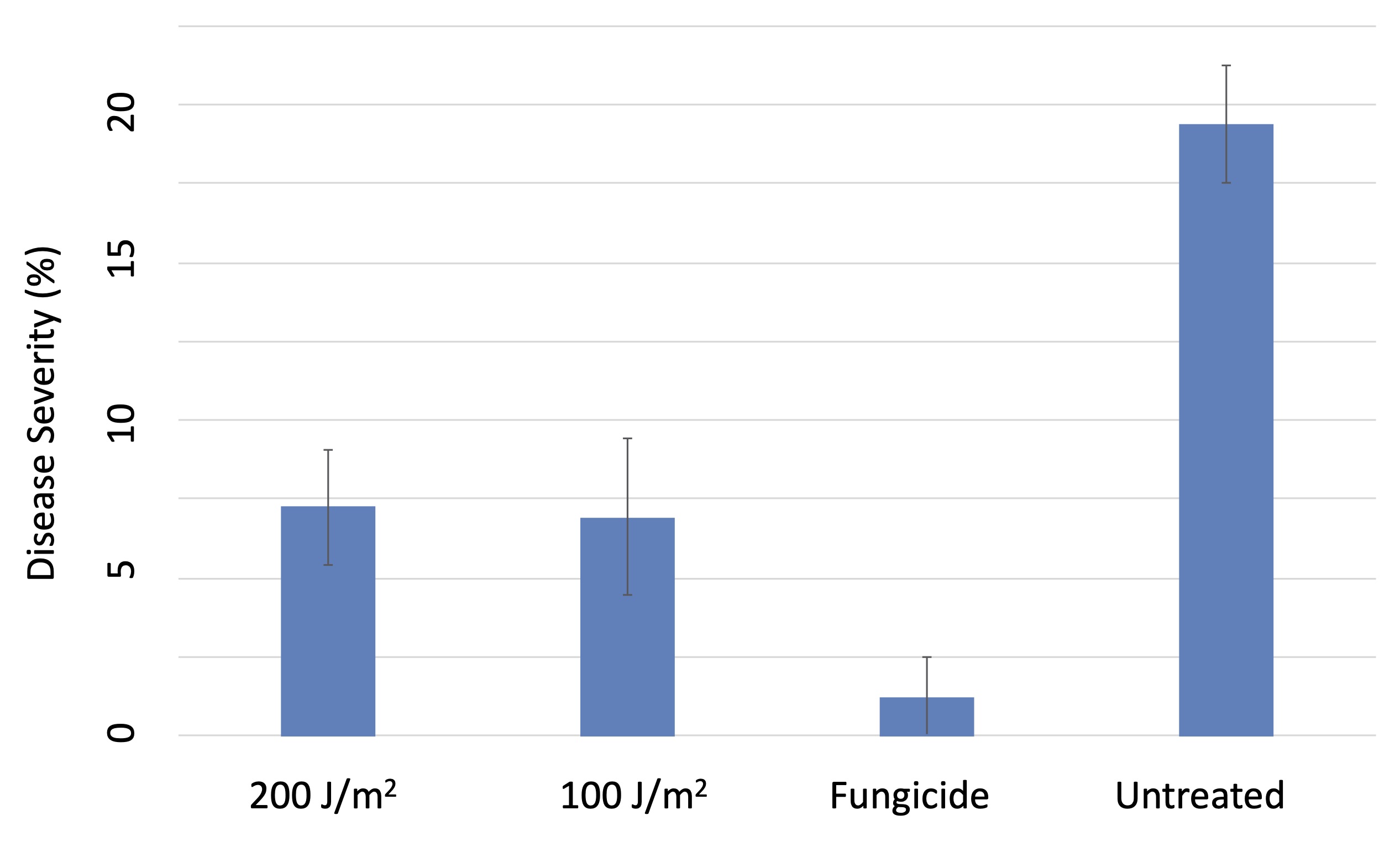
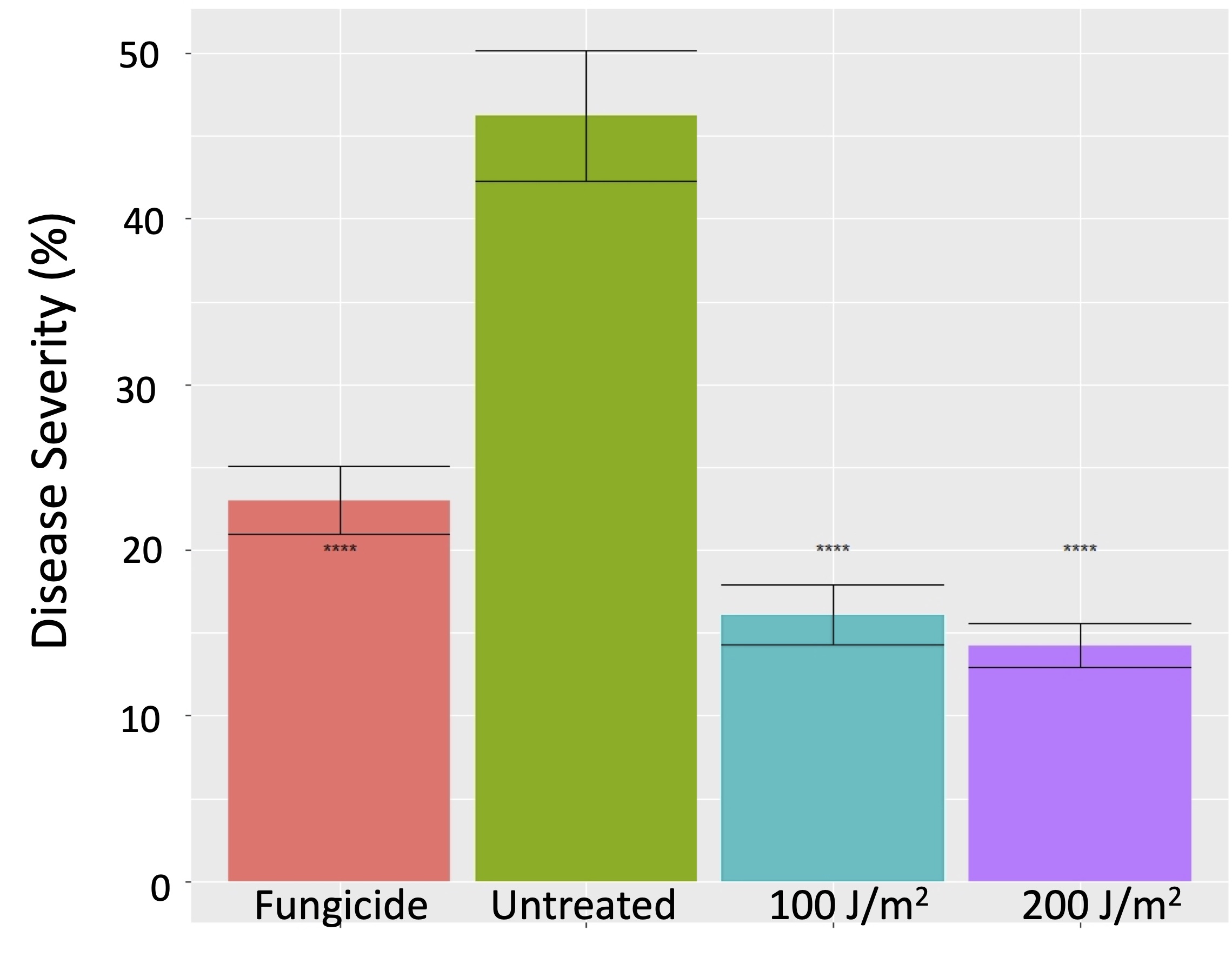
The 2019 trials produced another surprise: the UV treatments effectively suppressed sour rot (Figure 7). This disease is a complex mess involving bacteria, fungi and fruit-feeding insects. We still don’t understand how UV is accomplishing this reduction, but given that there are very few effective means to suppress sour rot, any efficacy due to UV treatments is worth further investigation.

In addition to suppressing plant pathogenic fungi, UV treatments can also suppress populations of phytophagous mites (Figure 8). A number of studies have noted that UVB and UVC treatments can kill eggs of spider mites and European Red Mites. In addition to these effects, our preliminary trials indicate that the UV treatments can also alter behavior of adult mites, reduce egg laying, and reduce fecundity of the generation of surviving mites that emerge from UV treated eggs.
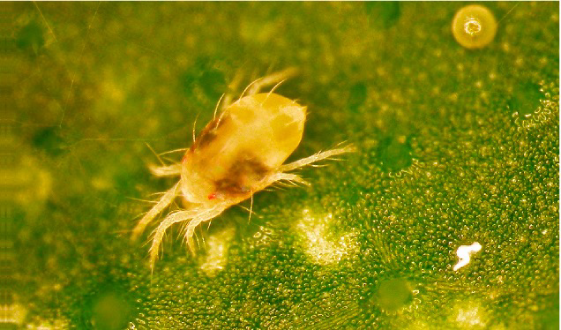
As in our strawberry work, we eventually wanted to adapt the tractor-drawn grape UV array to a robotic carriage, and our partnership with SAGA robotics made this possible (Figure 9). The navigation autonomy of the SAGA robot (Thorvald) is capable of tracking within a few centimeters of the trellis center at operational speeds between 1.25 to 2.5 mph. We evaluated UV doses between 100 J/m2 and 200 J/m2 at frequencies of either once weekly or twice weekly. All of the evaluated doses significantly suppressed powdery mildew on both fruit and foliage, and the twice-weekly 200 J/m2 treatment provided control that was superior to the fungicide standard (Figure 10).
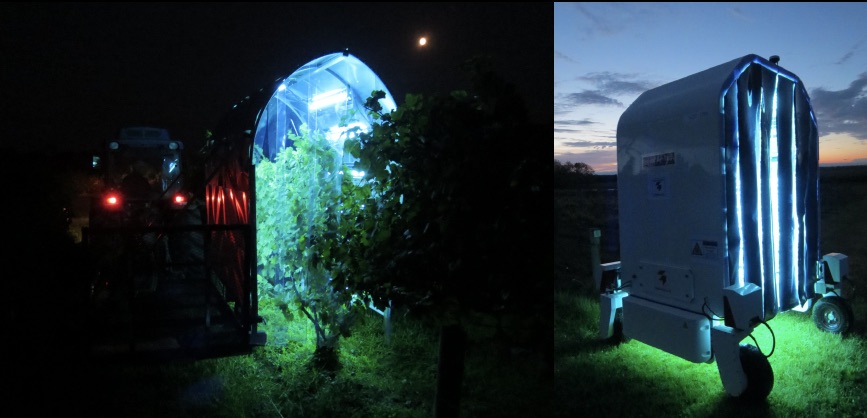
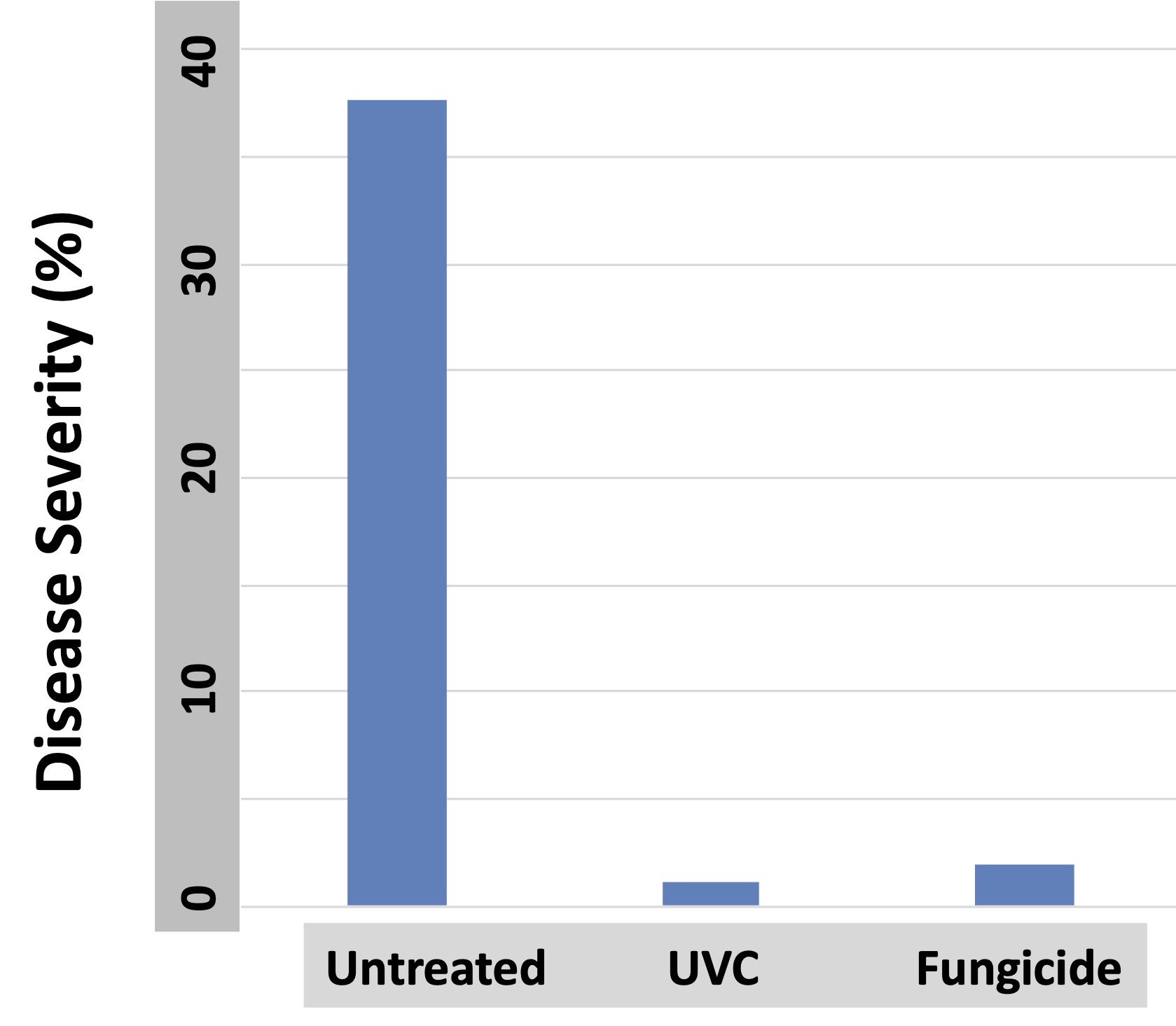
What’s Next?
We are collaborating with growers and scientists at multiple locations in the U.S. and Europe, including Bully Hill Vineyards in Hammondsport, N.Y.; Washington State University’s research and extension center in Prosser, and the USDA Horticultural Crops Research Center in Corvallis, Ore. as well as multiple locations in California, with designs and materials for UVC lamp arrays adapted for their vineyard pruning and training systems. These trials will be conducted over the course of the 2021 growing season. More about the autonomous robot Thorvald can be found at sagarobotics.com/. Our international working group is described on our project website: LightAndPlantHealth.org. It is a large, multidisciplinary, multi-institutional and international group representing several U.S. and overseas universities and government agencies, with industrial partnerships (Figure 11).
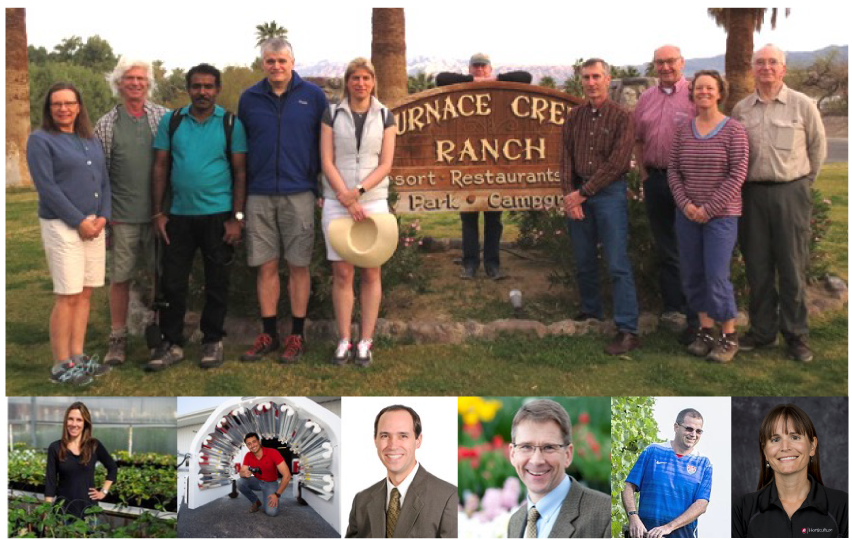
The design of a lamp array to match a particular crop canopy and target pest biology is a critical aspect determining of the success of the treatments. Our cooperative projects with growers across the US have always involved our array designs and electronics. Some growers have designed and fabricated the various carriages for the arrays. But the UV array itself is NOT a DIY project, nor is calibration and the photobiological and epidemiological calculations that enter into calculations of a proper UV dose for specific applications. In addition to the engineering and biological considerations, both UVB and UVC can be injurious to you unless devices are properly designed and the lamps are properly shielded from direct view. No person should ever have an unshielded view of germicidal UV lamps, as there is a significant risk of eye and skin damage from exposure UVB and UVC. The protective gear that is required for safe applications is not expensive, and consists of UV-opaque clothing that covers all exposed skin, disposable gloves and a face-shield and eye protection rated for protection from UV. The arrays shown in this article also incorporate clear PVC curtains at each end of the array to limit escape of UV from the array. As would be the case with any IPM technology, UV does not pose undue risks to operators or the environment if used properly. Proper training and use protocols are the key to safe and effective applications.
Our work has been funded by competitive grants from the USDA Organic Research and Extension Initiative, and the USDA Specialty Crops Research Initiative. Additional support has been provided by the National Research Council of Norway, the New York Farm Viability Institute, the USDA Sustainable Agriculture Research and Extension Program and Bully Hill Vineyards. We work as a diverse international group to promote this research area and its applications, and to act as a resource to train others. The work spans disciplines from plant growth and photobiology to physics and lighting technology.
David M. Gadoury is a senior research associate in Cornell’s Plant Pathology and Plant-Microbe Biology Section at Cornell AgriTech, where his program focuses on pathogen ecology, pathogen biology and disease management. He leads the Light and Plant Health Group.
References
Gadoury, D.M., Pearson, R.C., Seem, R.C., Henick-Kling, T., Creasy, L.L., and Michaloski, A. 1992. Control of diseases of grapevine by short-wave ultraviolet light. Phytopathology 82:243.
Janisiewicz, W. J., Takeda, F., Glenn, D. M., Camp, M. J., & Jurick, W. M. (2016a). Dark Period Following UV-C Treatment Enhances Killing of Botrytis cinerea 3.Conidia and Controls Gray Mold of Strawberries. Phytopathology, 106(4), 386–394. https://doi.org/10.1094/PHYTO-09-15-0240-R
Michaloski, A.J. 1991. Method and apparatus for ultraviolet treatment of plants. U.S. Patent no. 5,040,329.
Onofre, R. B., Gadoury, D. M., Stensvand, A., Bierman, A., Rea, M., and Peres, N. A. 2019. Use of ultraviolet light to suppress powdery mildew in strawberry fruit production fields. Plant Dis. 105:0000-0000 (in press).
Suthaparan, A., Stensvand, A., Solhaug, K. A., Torre, S., Mortensen, L. M., Gadoury, D. M., Seem, R. C., and Gislerød, H. R. 2012. Suppression of powdery mildew (Podosphaera pannosa) in greenhouse roses by brief exposure to supplemental UV-B radiation. Plant Dis. 96:1653-1660.
Suthaparan, A., Stensvand, A., Solhaug, K. A., Torre, S., Telfer, K. H., Ruud, A. K., Mortensen, L. M., Gadoury, D. M., Seem, R. C., and Gislerød, H. R. 2014. Suppression of cucumber powdery mildew by supplemental UV-B radiation in greenhouses can be augmented or reduced by background radiation quality. Plant Dis. 98:1349-1357.