Over the past several years, few irrigated regions of the country have escaped the impacts of drought and in many places this has translated to increased costs and reduced availability of irrigation water. California growers in particular have experienced dramatic reductions in surface water deliveries and have increasingly turned to using groundwater to make up the surface water shortfall. Consequently growers have experienced unprecedented water table level reductions that have increased the price of pumping and maintenance on their water wells. Growers have also responded to the drought by increasing personal and personnel resources dedicated to water management and have increased their efforts to better understand the complexities of irrigation water management. Efforts to improve on-farm water management practices must include these key elements:
- Elevating irrigation system design and management expectations.
- Better exploit our understanding of crop development and physiology including crop sensitivity to water stress.
- Increasing our capacities to measure and manage soil water storage.
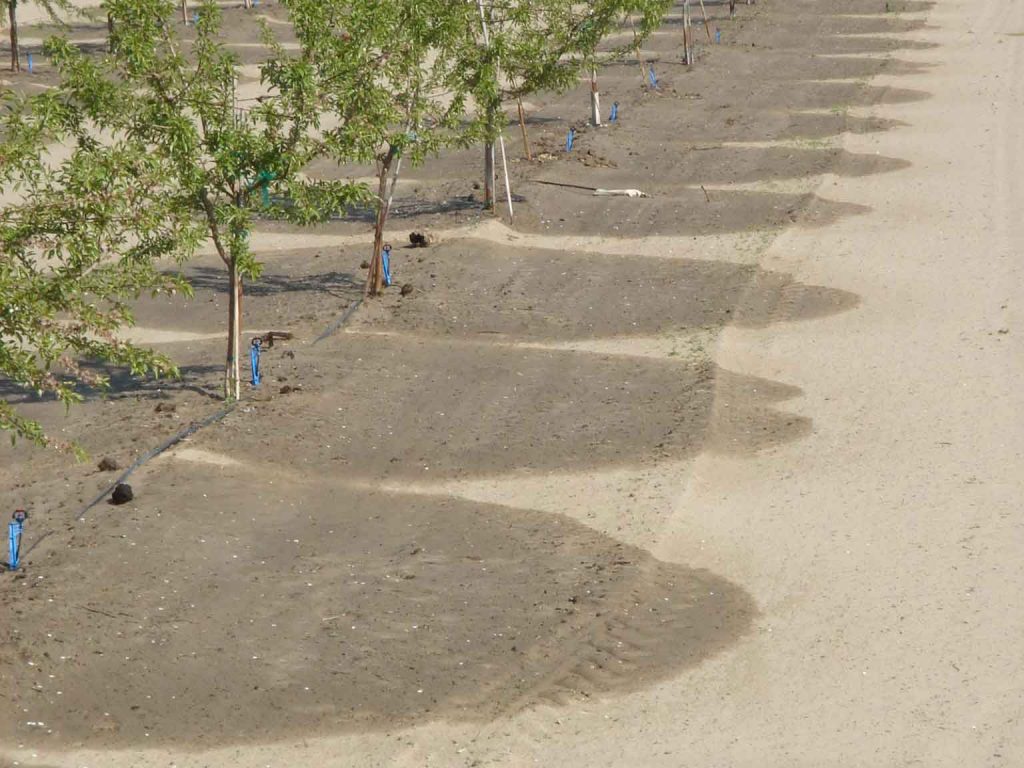
Proper evaluation and integration of these key water management elements can be complex but can result in operating whole farm and field irrigation systems at peak efficiency over time. For example, it may not help us to increase the monitoring and measurement soil moisture if we do not have a more complete understanding of how specific changes in soil moisture content influence crop water stress and crop performance. Similarly it would not be difficult to misinterpret plant water stress or soil moisture information in a field where water is not applied in a uniform manner. And how might field indicators of soil water availability be used in different field management settings? Interpreting soil moisture readings in a drip irrigated field that applies water multiple times per week will differ from that of a furrow or flood irrigated field that is irrigated using two to three week intervals. These complexities help to point out that each field is unique from a water management standpoint and that irrigation decision makers should not rely too heavily on any one piece of information to guide their water management program without also considering broader systems information.
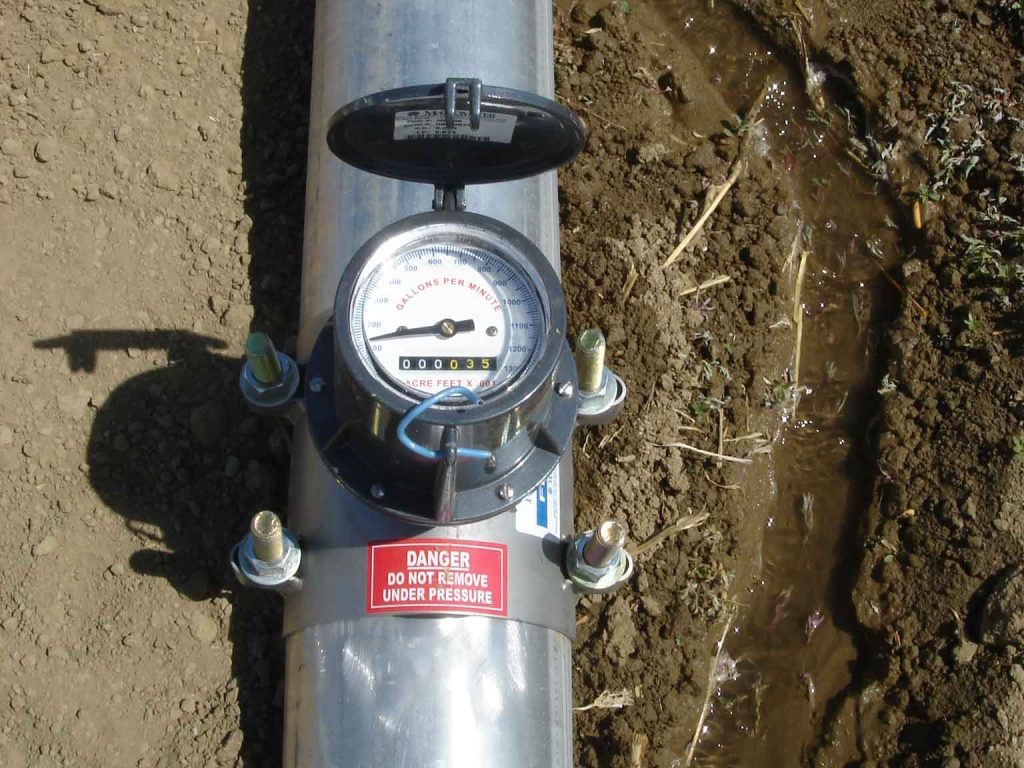
Irrigation system design and management
Improperly designed irrigation systems are incapable of achieving high performance levels making efficient water management an impossibility regardless of how well water might be scheduled. Application efficiency is a fundamental measure of irrigation system performance defined as the amount of beneficially applied water in relation to the amount of total irrigation water applied to the field. One of the biggest obstacles to developing field systems with high application efficiency comes from the fact that many irrigation systems do not apply water uniformly. Water applied in a non-uniform manner which commonly leads to larger soil water deficits on low water application areas and over-irrigating areas of the field that have higher than average application rates. To maintain preferred soil moisture levels in all areas of the field, water managers typically compensate by over-applying water on portions of the field which causes losses to leaching or run-off both considered to be non-beneficial uses of water. Properly designed irrigation systems work to achieve a high degree of uniformity and deliver near equal amounts of water throughout the field.
One of the more challenging design issues in drip irrigation systems is achieving near uniform pressures throughout the field. Even when fitted with pressure compensating emitters, fields that have large differences in line pressure are susceptible to significant differences in emitter output causing non-uniform applications. This problem is more commonly associated with long field lengths which stretch the design capacities of our current drip irrigation products. And while many drip irrigation fields have relatively high distribution uniformity, maintenance and management issues continue to leave many fields with less than optimal efficiencies. Field lengths of less than 800 feet are less prone to this concern while it is a much more common issue in field lengths exceeding 1100 feet. This emphasizes the need to carefully consider the products being purchased and the pressure requirements of that product. Uniformity issues can also be exagerated as the system ages or in systems that are not properly filtered and maintained to avoid biological and mineral contamination.
Occasionally simple modifications in surface irrigation systems can also result in significant improvements in distribution uniformity and application efficiency. Many flood and furrow irrigated systems experience slow water advance times down the furrow or irrigated strip before the irrigation is completed resulting in long infiltration periods at the head end of the field relative to the infiltration period at the lower end of the field resulting in higher amounts of applied water at the head end of the field. Solutions to this problem have been achieved by increasing the on-flow rate of irrigation water, reducing the size of the irrigation check and by modifying the surface soil roughness to allow water to more freely move to the bottom of the field. These relatively modest system design changes can have a significant impact on delivering water with greater uniformity and efficiency.
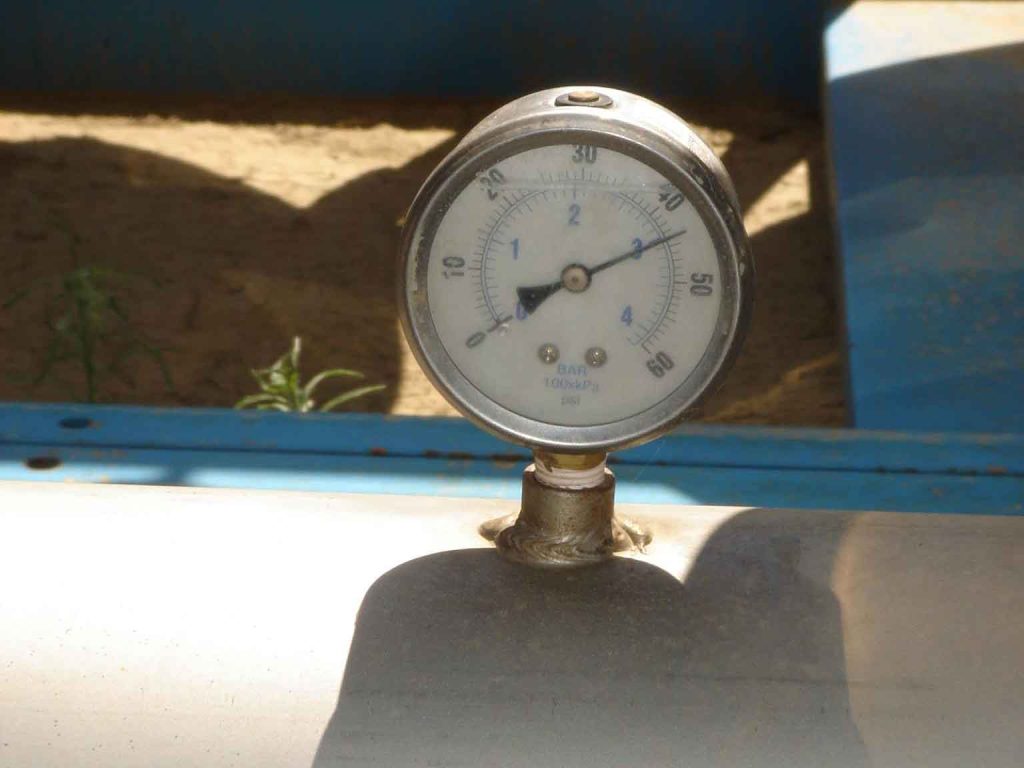
Crop development, physiology and water stress management
Crop sensitivities to water stress are not constant throughout the growing season depending on crop type, growth stage and atmospheric conditions. Most field and row crops are highly susceptible to the impacts of limited water availability during the germination and seedling development stages and require high soil moisture availability in the surface soil during this period. However, as the early vegetative growth period is initiated crops like cotton and small grains can go many weeks before the first seasonal irrigation water is required. This is partially due to the rapid root growth that occurs in these plants and soil water is more easily extracted during these low evapotranspiration days. Alternatively, many vegetable crops including tomatoes, carrots and the brassicas require more frequent irrigation events early season to relieve mild water stress during this period needed to support a rapidly expanding plant canopy. Later in the season when roots are well established and the lower portions of the soil profile are exploited, many deep-rooted crops are less sensitive to water stress and water management strategies can be incorporated that take advantage of deep soil water reserves resulting in delayed irrigation scheduling.
Similar issues can be observed with the permanent crops as early season root flushes occur at different intervals allowing soil moisture to be exploited at varied depths depending on crop type. Generally, crop water stress sensitivities in permanent crops are often more acute during early leaf out periods if winter rains have not fully charged the soil profile and again during the rapid fruit growth periods. Monitoring the developmental stage of the crop often provides insights to the physiological periods of the crop that are more sensitive to water deficits and points to periods when water deficiencies are more likely to result in impacts to yield and quality. Understanding these more sensitive water stress periods also allows us to better plan on the time of year to increase crop water stress monitoring by using tools such as the pressure chamber or canopy temperature tools that are used to evaluate the relative degree of crop stress. And while water stress limits may differ during the growing season, using established water stress guidelines when available, can provide sound guidance on irrigation scheduling decisions.
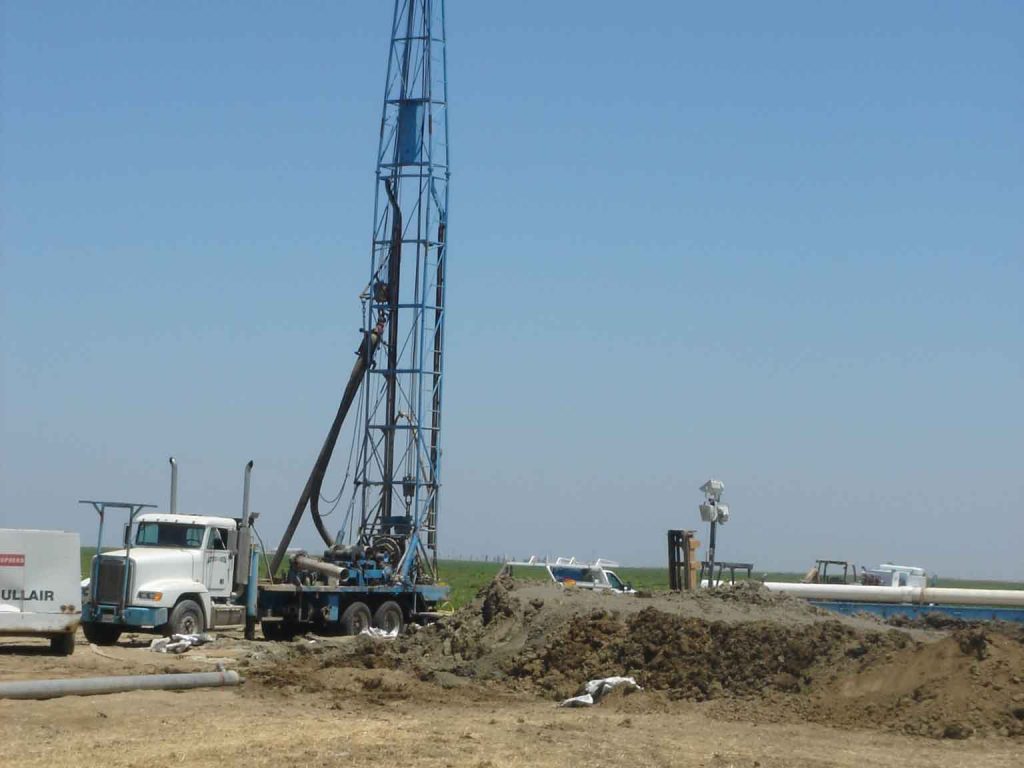
Managing and measuring soil water storage
The measurement of soil water can appear to be an uncomplicated process that involves placing soil moisture sensors in the soil and reading the values to provide an irrigation management decision. And while this may be satisfactory for relatively simple, uniform and well understood field systems, there is much that should be considered in developing an approach to soil moisture monitoring if the goal is to maximize the beneficial uses of applied irrigation water. Successful growers and consultants that regularly monitor soil water and use the information as an irrigation scheduling tool agree that there are several important questions to consider before purchasing, installing and using the sensors to make informed irrigation decisions. Before investing the time and resources in soil moisture monitoring, establish basic achievable goals with the understanding that the higher the expectation and more detailed the goal, greater effort and resources will be required.
As goals are established for field monitoring, consideration of the type of sensor to be used is a good place to start as well as the price of those sensors and systems. Field soil moisture systems can start with an investment of a few hundred dollars or less and can run into several thousand dollars for a single monitoring site. Soil water sensors can monitor soil water content more directly or they can provide direct or indirect measures of soil water potential. Each sensor type can be useful depending on the goals and information needs established. Identifying a field location or locations and placing the sensors at appropriate depths is also important and care should be taken to select locations that are representative of soil water conditions in the crop root zone. Monitoring multiple soil depths can also provide information on soil water storage and percent soil profile water depletion level. Depending on the time of year, many growers have turned to using different soil depths as triggers to initiate irrigation events using sensors placed at more shallow depths to schedule early season irrigation events.
Evaluating the readings from soil moisture devices also requires some experience and understanding of soil water retention characteristics of the field being monitored. Although estimated values of field capacity, permanent wilting point and plant available water can be referenced for various soil textural classes, these values are very generic and can misrepresent actual site values that can be used for irrigation scheduling purposes making the information less reliable. Developing individual field or soil type data for your fields often aids in providing more specific and repeatable information that can improve irrigation decision making.
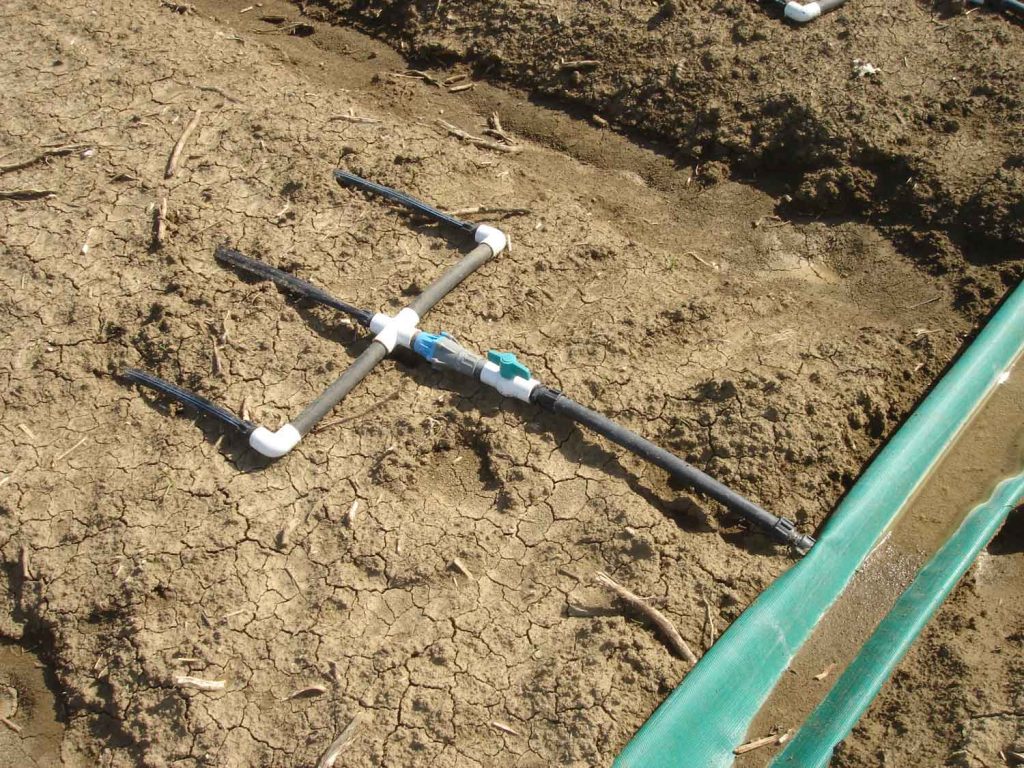
Integrating the information
Recognizing the need to integrate key water system information into a coherent field and farm water management plan system requires work and experience in evaluating multiple system elements and will assist in avoiding the tendency to place the focus and reliance on singular management indicators. Integration of these primary water management elements will have impacts on the time and amount of field applied water and can have significant impacts on farm water use by limiting the application of water that does not directly benefit the crop. Recognizing the importance of integrating appropriate information from multiple sources to manage farm water requires that we begin using the tools available to us to document and interpret a wide variety of information of our irrigation systems, soil systems and our individual cropping systems.
Independent evaluations of an irrigation system performance can provide feedback on the current issues of concern for individual systems and can provide an assessment of whether system maintenance is badly needed or if help determine if the system requires improved pressure regulation, higher flow rates or other design modifications to operate more optimally. Water applied uniformly and with high efficiency limits water and nutrient losses to the groundwater and results in more uniform crop yield and quality. But information related to application efficiency or distribution uniformity can also aid in targeting locations for soil moisture monitoring sites and locating sites to monitor plant stress and crop growth.
Using available knowledge of plant development and physiology can aid in identifying periods when the crop is particularly sensitive to water stress events and aid in irrigation scheduling decisions by either limiting the drawdown of soil moisture reserves during those periods or by extending the irrigation cycle. In a similar manner, the use of plant water stress indices can be used to hasten or delay irrigation events and help establish the intensity and duration of water stress events. Numerous university publications are available that provide sound information on the development and physiology of specific crops. This information often includes water management studies that can provide tools to identify crop developmental stage as well as information on water stress sensitivity and periods of relative tolerance to water stress.
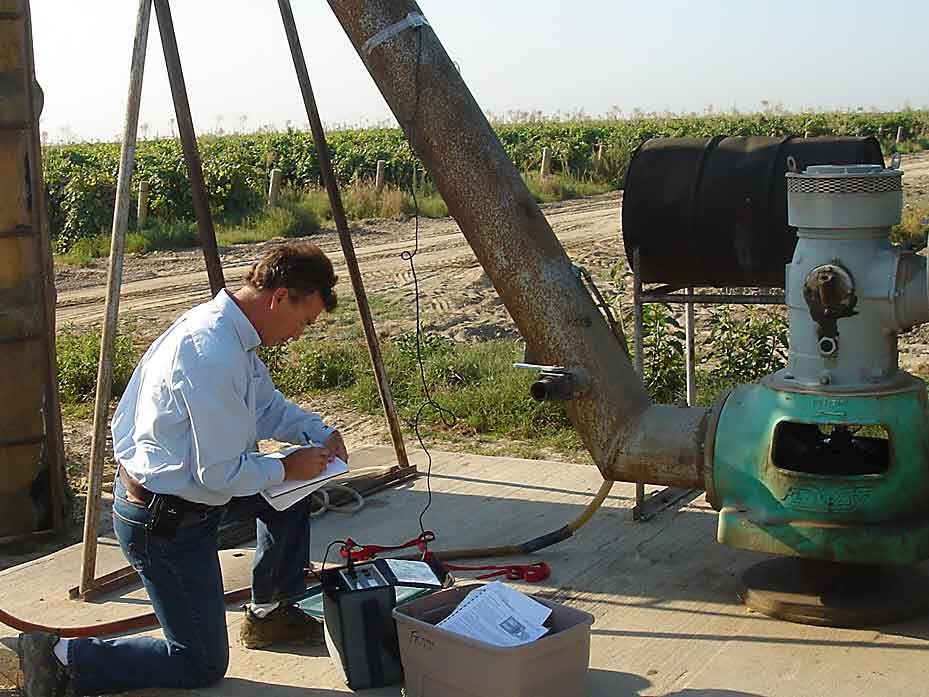
Establishing reasonable goals and expectations for monitoring soil water status and using soil sensor information for irrigation decision making purposes can assist in tightening irrigation schedules thereby reducing the likelihood of excessive losses while also reducing the risk of crop losses that result from elevated crop water stress levels. This information is particularly useful when combined with other irrigation scheduling tools such as crop evapotranspiration estimates and crop water stress indicators.
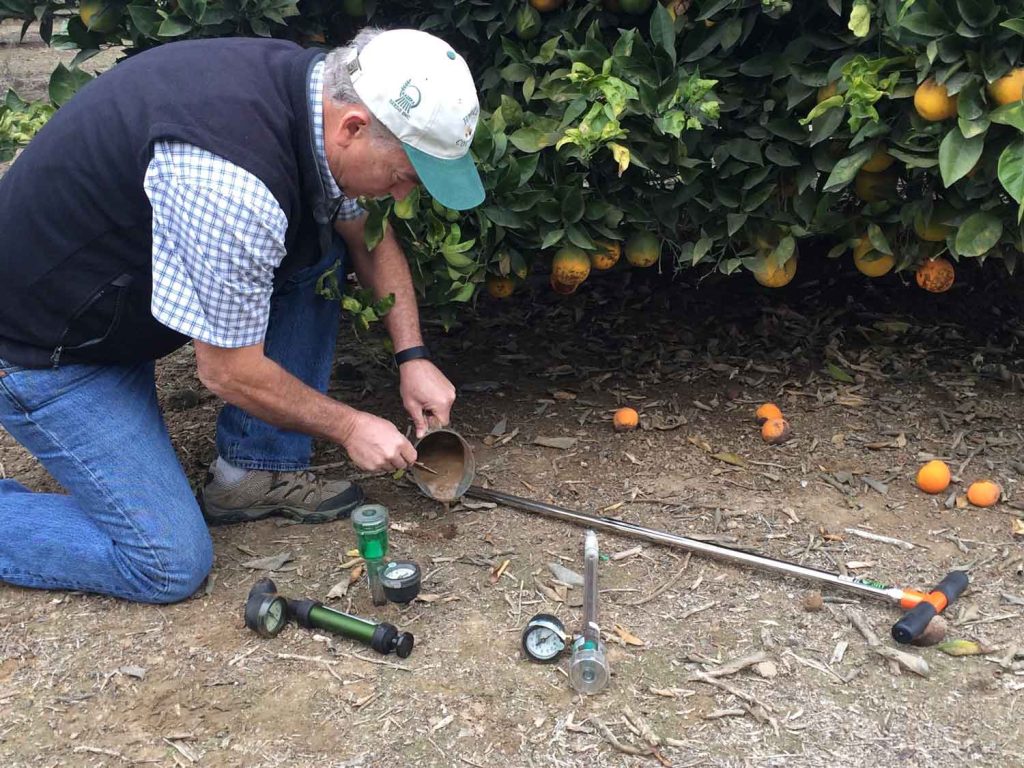