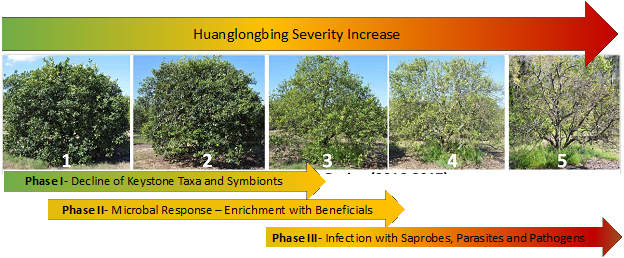
The world population is projected to reach about 10 billion individuals by 2050. As a result, the agricultural sector needs to produce more food despite several looming challenges, including the shrinking of arable land, erratic and extreme weather patterns and pest and disease outbreaks. Higher agricultural outputs per surface basis is one key element to solve the equation of a growing demand, which inevitably results in an increase of agrochemical inputs (pesticides, fertilizers). The global agrochemical market size is valued at $235.2 billion in 2023 and is expected to reach $280 billion by 2028, growing at a compound annual growth rate (CAGR) of 3.7% during the forecast period. The major driving force behind this uptrend comes from the organic sector. Hence, the global agricultural biologicals market (biopesticides, biostimulants and biofertilizers) is projected t
grow at a CAGR of 13.8% to reach $27.9 billion by 2029 from $14.6 billion in 2023.
There is a growing consumer demand for organically grown and safe agricultural products. There is also a collective awareness of the potential risks associated with pesticide use, including impact on human health (e.g., increase of cancers linked to pesticide use), contamination of environmental resources (e.g., leaching of chemicals in groundwater) and non-target side effects on wildlife (e.g., bee population collapse). This has led to a tightening of policies by governments and regulatory agencies for conventional chemical use and chemical residue limits and the phasing out of harsh synthetic agrochemicals. Growers are at the forefront of the sustainable ag movement and have adapted their farming practices to meet both consumer and agency demands. Replacing synthetic chemicals with organic products or biologicals in their agrochemical arsenal can be challenging because of the limited scientific information on their mode of action and range of efficacy. In this article, we will mostly focus the discussion on sustainable strategies to control diseases with biopesticides, the discovery pipeline within the framework of my research and the prospects for the future.
In Integrated Pest Management
Fostering sustainable farming practices that promote and enhance agroecosystem health and increase biodiversity are instrumental to our long-term success. Integrated pest and disease management programs (IPM or IPDM) have been central to this philosophy. The framework of IPM programs is to reduce synthetic agrochemical inputs and rely first on alternative strategies, such as adapted management practices, use of resistant/tolerant plant varieties and deployment of organic agrochemicals and biologicals. IPM programs were at times challenging to implement to manage certain crop diseases because of the lack of relevant alternatives to synthetic agrochemicals. Organic agrochemicals suffer from a short window of activity and under high disease pressure they must be applied often. Also, those products are not free of environmental risks. For example, the copper-based Bordeaux mixtures have been used for over a century in viticulture and are known to impoverish vineyard soil biota and affect aromas in wine.
Biologicals also often suffered from a lack of credibility because of their inconsistency to control diseases. From a grower standpoint, there is too much economical risk to rely on uncertainty. However, recent scientific discoveries and technological advances have helped strengthen IPM programs in many ways. First, they have and continue to improve the formulations of agrochemicals (conventional, organic and biological) and, most importantly, their deliveries to target pests and pathogens. Scientific reports indicated that only 0.1% to 1% of sprayed material reaches its target depending on the system. Thus, increasing delivery efficiency will reduce leaching of chemicals in the environment. Second, the advent of ‘omics’ technologies and affordability of sequencing costs have allowed industry to identify biological candidates in a quick and reliable manner. This has revolutionized the field of microbiology (among others) that traditionally used ancestral culturing techniques to single out biological agents from environmental samples, which equated to finding a needle in a haystack. In today’s era, we can generate large-sequence datasets that capture not only entire microbial communities associated with plants or environments but also shed light on their biological functions. To be able to see in a high-throughput manner ‘who does what’ has streamlined the discovery of biological products.
Finding Beneficial Microbes for Diseased Systems
My lab has been using omics tools to identify beneficial organisms inhabiting citrus and grapevine that could be leveraged to manage Huanglongbing (HLB) and Pierce’s disease (PD), respectively. Both diseases are caused by bacterial pathogens transmitted by insect vectors following feeding on the plant host. In citrus HLB, the bacterium (Candidatus Liberibacter asiaticus) lives in the phloem tissues that transport sugars downwards, from leaves to fruits and roots. In grapevine, the bacterium (Xylella fastidiosa) lives in the xylem tissues that transport water and nutrients upwards from roots to leaves. In both pathosystems, the buildup of bacteria in the hosts’ vasculature leads to disruption of channel transport to a point that becomes detrimental to the plant. Citrus and grapevine decline can happen in as quickly as two years, but in some cases, the hosts can sustain the infection for several years. Our hypothesis is the microbial communities living with the host provide protection against the pathogens with the goal of leveraging their benefits for crop protection.
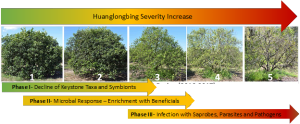
A survey of citrus orchards in Florida and sequencing of the microbial communities associated with roots of trees with a range of disease symptoms revealed interesting information on the disease etiology (Figure 1). Our results showed there was an initial decline of keystone taxa (native organisms that play a role in the stability of an ecosystem) and symbionts such as mycorrhizae fungi for trees that contracted the disease (Phase I). This was followed by a microbe-mediated response to infection, with enrichment of several beneficial organisms that have the capabilities to stimulate the tree immune response or provide direct antibiosis to pathogens (Phase II). In the late phase of the infection (Phase III), we measured an increase of soilborne parasites and pathogens (Fusarium, Phytophthora) and saprophytic fungi that decompose decayed roots. Those findings indicate either HLB made trees more vulnerable to root pathogens or vice versa, but in either case, this synergistic effect caused trees to decline at a faster pace. This research led to the pursuit of two strategies that are now being evaluated to combat HLB. The first strategy is to re-introduce into the system beneficial microbes or microbial natural products isolated from Phase II and verify these can support tree immunity. The second strategy is to identify cultural practices like soil amendments that support the keystone and symbiotic communities.
Similar to citrus, we surveyed vineyards and profiled the microbiome of grapevine with a range of Pierce’s disease symptoms (Figure 2). Here, we found interesting data when looking at the vine lignified shoot tissues (canes). In comparison to the root system, there are very few microbes living in the plant vasculature and even more so in annual tissues like canes that are pruned off every year. This is a perfect environment for the pathogenic bacterium to thrive due to the limited microbial competition. Our results showed that, just like in citrus roots, there was a microbial-mediated response to infection and that two bacteria that also inhabit the vascular system correlated negatively with the pathogen Xylella fastidiosa. In other words, when those two beneficial bacteria were present and abundant, the pathogenic bacterium was low, and vines were healthy. Our group further re-isolated those beneficial bacteria and confirmed in greenhouse bioassays that when they were inoculated to grapevine, they provided protection against Pierce’s disease. These biological control agents are currently being evaluated at UC Davis in field trials. UC patented those technologies, and we are partnering with the private sector to develop injectable or sprayable products that could be commercialized for Pierce’s disease management.
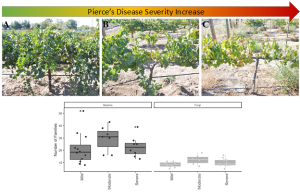
There is a push from agricultural commodities to replace synthetic agrochemicals with environmentally friendly solutions. There are a lot of incentives for agrochemical companies to develop bio-based products, including an easier path to EPA registration. The lower residual toxicity levels of biopesticides make them the perfect choice to meet stringent environmental standards and address food safety and quality. However, biologicals still suffer from higher production costs because they are not produced and distributed in high volumes like their synthetic chemical counterparts. Increasing scalability and improving consistency in efficacy are two major hurdles for biopesticides to gain larger market share in the future.
References
Ginnan et al. 2020. Phytobiomes. https://doi.org/10.1094/PBIOMES-04-20-0027-R
Deyett and Rolshausen 2019. Frontiers in Plant Science. https://doi.org/10.3389/fpls.2019.01246