Plant-associated arbuscular mycorrhizal fungi (AMF) participate in soil carbon storage, improve soil aggregation and promote plant health and crop yield. Like other perennial crops, citrus trees create associations with AMF (Wu and Srivastava 2017; Xi et al. 2022) which have been shown to improve crop nutrition (Wu and Zou 2009), enhance tolerance against abiotic stressors like drought (Wu et al. 2019) and induce better root development (Wu et al. 2012). Due to multiple benefits of AMF to soil and plant health, AMF has gained much attention, leading to a rapidly expanding market in mycorrhizal biostimulants focused on improving crop yield and root development of horticultural crops (Igiehon and Babalola 2017; Chen et al. 2018).
In agricultural systems, abundance of AMF can be negatively impacted by intensive cultivation, leading to a decrease of AMF spores and infective mycelium; thus, native AMF are often promoted by cover cropping and reducing soil disturbance (Bowles et al. 2016b). AMF inoculation can be successful in soils with limited native AMF, poor soil health and low productivity (Verbruggen et al. 2012; Rog et al. 2025). Our study aimed to investigate the effects of inoculated and uninoculated triticale cover crops on soil health and carbon storage in the alley and tree rows of a commercial lemon orchard in the Californian Central Coast region.
Experimental Design and Soil Analysis
The study was conducted at a commercial citrus orchard located in San Luis Obispo County between fall 2019 and spring 2023. The testing site was a 6-acre block planted with Citrus limon (L.) Burm. f. ‘Lisbon Lemon’. The experimental design was a randomized complete block design with three blocks and three treatments in each block. Treatments included a control (bare fallow with no herbicide application), a cereal cover crop (Triticale (Secale x Triticum L.) drill-seeded at 110 pounds per acre and a cereal cover crop inoculated with AMF (110 pounds per acre inoculated with commercial AMF inoculum Rhizophagus intraradices, 300 propagules per gram at 10 pounds per acre). Cover crops were seeded in alley rows every growing season from 2020 to 2022. The timing of cover crop seeding and AMF inoculation was selected in accordance with winter rain events. In winter 2022-23, early rain promoted germination of cover crop seed from previous years and no AMF inoculation was applied. Cover crops were completely rain-fed, with no supplemental irrigation during dry months, and were mowed in June each year.
In the first three years, soil samples were collected between trees from the tree rows and from the center of the alley row at 0 to 6 inches and 6 to 18 inches depth. In year four, soil sampling was adjusted to better understand the link between the position on the orchard floor, microbial community structure and soil carbon dynamics as affected by the cover crops and AMF inoculation. Composite soil samples were taken from each plot at four functional locations defined as follows: between two trees on a berm (Location 1), the transition section where the berm ends but no cover crop is grown (Location 2), the cover crop edge (weeds in control plots) (Location 3) and the center of the alley row (Location 4; Fig. 1). Fresh soil subsamples were sent to Ward Laboratories for soil microbial community structure analysis using phospholipid fatty acid (PLFA) and neutral lipid fatty acid (NLFA). Soil samples were sieved, air dried and analyzed for total soil C (%), permanganate oxidizable carbon (POXC) and mineralizable carbon (Min C). Min C, also referred to as soil respiration, is an indicator of microbial activity, while POXC, also referred to as active carbon, can be considered a microbial food source. Both Min C and POXC are commonly used as indicators of soil health (Moebius-Clune et al. 2016; Norris et al. 2020).
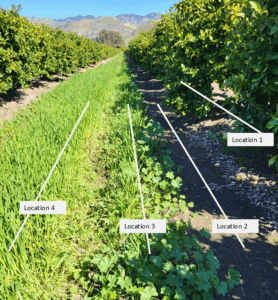
The Overlooked Role of Weeds and Native AMF.
After two years of treatment implementation, there was no effect of cover crops or inoculation with AMF on the total soil C, POXC and Min C in the tree or alley row (data is not shown). Likewise, there was no treatment effect on total soil C, POXC and Min C at any of the four locations across the orchard floor after three years of treatment implementation (Table 1). Our control treatment had weeds (predominantly Malva and some filarees during soil sampling) which added C sources to soil, likely causing the lack of a significant difference between cover crop treatments and control.
Our PLFA and NLFA microbial biomass data showed no significant persistent inoculation impact on the microbial community compared to the non-inoculated cover crop and the weedy control treatment plots after three years of treatment implementation. We did not observe any differences in microbial biomass between the cereal cover crop and the weedy control treatment, indicating weeds supported the microbial community similarly as the cereal cover crop (Fig. 2).
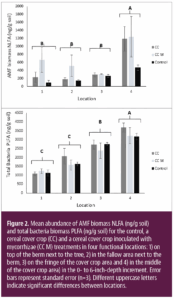
The lack of effect of the inoculated cover crop compared to the non-inoculated cover crop and control treatment on soil C metrics and microbial community structure in our study may be attributed to native AMF species forming associations with plants growing in the alley row and leading to similar results as those observed in the inoculated plots (Wilson et al. 2009; Bowles et al. 2016a,b; Agnihotri et al. 2021; Lin et al. 2023). These findings suggest native AMF can be successfully promoted in the alley rows by weeds or a cover crop and may provide a more effective strategy than inoculation in perennial citrus orchards. Therefore, AMF inoculations may not add value in soil with existing plant cover and low soil disturbance.
Cover Crop Effects Extend Beyond Area Directly Underneath Plant Cover
In contrast to treatment comparisons, soil C indicators showed greater values in the alley rows compared to the tree row (Table 1). In the topsoil, the cereal cover crop, weedy control and repeated additions of tree prunings in the alley row supported more microbial biomass, including AMF (Fig. 2), and had higher C storage potential compared to the tree row that had less plant matter input in the top soil and potentially experienced C loss due to pulses of C mineralization during dry-wet cycles associated with frequent irrigation (Lundquist et al. 1999; Denef et al. 2001; Lopez-Sangil et al. 2018). The location effects in this orchard trial suggest different managent of trees and alley rows leads to soil heterogeneity across the orchard floor.
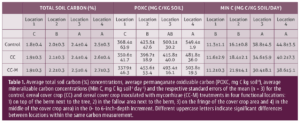
The management in the alley rows more positively impacted soil C cycling indicators and soil health with some carry-over from the cover cropped alley rows into adjacent areas. More specifically, total C and POXC concentrations declined from the center of the alley rows to the area next to the citrus trees (Table 1). In addition, total C and POXC were greater in location 2, which represents the plant-free area adjacent to the cover crop and impacted by wheel traffic, compared to location 1 immediately next to the citrus tree. An orchard floor gradient assessment from the center of the cover cropped row toward the tree row with bare soil showed a potential carryover effect from the cover crop row into the fallow area between the trees and the edge of the cover crop. Citrus trees may potentially receive more nutrients outside of the berm by extending lateral roots into alley rows and by making associations with AMF that have extensive hyphal networks. Therefore, lemon trees may benefit from soil improvements from cover crops grown in the alley row.
Mycorrhizae Affect Carbon Cycling through Recruitment of Bacteria
We used correlation analysis to evaluate the relationships among microbial groups and C cycling indicators (Table 2). In our dataset, AMF and saprophytic fungal biomass were positively correlated with total bacterial biomass (Table 2), which supports previous findings on the cooccurrence of AMF or saprotrophic fungi with bacteria in soil niches (Yuan et al. 2021; Zhang et al. 2022). We also observed no correlation between NLFA biomass of AMF and saprotrophic fungi. These findings suggest both AMF and saprotrophic fungi are able to recruit bacteria but the two groups of fungi occupy different soil niches. Correlation assessment of linking AMF NLFA biomass with soil C indicators showed no direct significant impact of AMF on soil C storage. In contrast to AMF, bacterial biomass was significantly correlated with all soil C indicators supporting studies where bacterial community was a major contributor to soil organic C accumulation (Zhang et al. 2020; Guo et al. 2021; Hu et al. 2023). As such, our findings suggest AMF may have an indirect influence on soil C dynamics by promoting bacterial biomass. Therefore, management practices that promote AMF, such as cover crops and reduced soil disturbance, help build soil organic matter and store carbon.
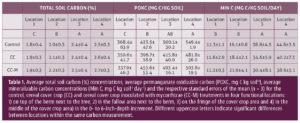
References
Agnihotri, R., A. Bharti, A. Ramesh, A. Prakash, and M.P. Sharma. 2021. Glomalin related protein and C16:1ω5 PLFA associated with AM fungi as potential signatures for assessing the soil C sequestration under contrasting soil management practices. European Journal of Soil Biology 103: 103286. doi: 10.1016/j.ejsobi.2021.103286.
Bowles, T.M., F.H. Barrios-Masias, E.A. Carlisle, T.R. Cavagnaro, and L.E. Jackson. 2016a. Effects of arbuscular mycorrhizae on tomato yield, nutrient uptake, water relations, and soil carbon dynamics under deficit irrigation in field conditions. Science of The Total Environment 566–567: 1223–1234. doi: 10.1016/j.scitotenv.2016.05.178.
Bowles, T.M., L.E. Jackson, M. Loeher, and T.R. Cavagnaro. 2016b. Ecological intensification and arbuscular mycorrhizas: a meta-analysis of tillage and cover crop effects. Journal of Applied Ecology 54(6): 1785–1793. doi: 10.1111/1365-2664.12815.
Chen, M., M. Arato, L. Borghi, E. Nouri, and D. Reinhardt. 2018. Beneficial services of arbuscular mycorrhizal fungi – From ecology to application. Frontiers in Plant Science 9. https://doi.org/10.3389/fpls.2018.01270.
Denef, K., J. Six, H. Bossuyt, S.D. Frey, E.T. Elliott, et al. 2001. Influence of dry–wet cycles on the interrelationship between aggregate, particulate organic matter, and microbial community dynamics. Soil Biology and Biochemistry 33(12): 1599–1611. doi: 10.1016/S0038-0717(01)00076-1.
Guo, Z., X. Zhang, J.A.J. Dungait, S.M. Green, X. Wen, et al. 2021. Contribution of soil microbial necromass to SOC stocks during vegetation recovery in a subtropical karst ecosystem. Science of The Total Environment 761: 143945. doi: 10.1016/j.scitotenv.2020.143945.
Hu, Q., T. Jiang, B.W. Thomas, J. Chen, J. Xie, et al. 2023. Legume cover crops enhance soil organic carbon via microbial necromass in orchard alleyways. Soil and Tillage Research 234: 105858. doi: 10.1016/j.still.2023.105858.
Igiehon, N.O., and O.O. Babalola. 2017. Biofertilizers and sustainable agriculture: exploring arbuscular mycorrhizal fungi. Appl Microbiol Biotechnol 101(12): 4871–4881. doi: 10.1007/s00253-017-8344-z.
Lin, J.S., M.V.M. Sarto, T.L. Carter, D.E. Peterson, C. Gura, et al. 2023. Soil organic carbon, aggregation and fungi community after 44 years of no-till and cropping systems in the Central Great Plains, USA. Arch Microbiol 205(3): 84. doi: 10.1007/s00203-023-03421-2.
Lopez-Sangil, L., I.P. Hartley, P. Rovira, P. Casals, and E.J. Sayer. 2018. Drying and rewetting conditions differentially affect the mineralization of fresh plant litter and extant soil organic matter. Soil Biology and Biochemistry 124: 81–89. doi: 10.1016/j.soilbio.2018.06.001.
Lundquist, E.J., L.E. Jackson, and K.M. Scow. 1999. Wet–dry cycles affect dissolved organic carbon in two California agricultural soils. Soil Biology and Biochemistry 31(7): 1031–1038. doi: 10.1016/S0038-0717(99)00017-6.
Moebius-Clune, B.N., D.J. Moebius-Clune, B.K. Gugino, O.J. Idowu, R.R. Schindelbeck, et al. 2016. Comprehensive Assessment of Soil Health – The Cornell Framework Manual.
Norris, C.E., G. Mac Bean, S.B. Cappellazzi, M. Cope, K.L.H. Greub, et al. 2020. Introducing the North American project to evaluate soil health measurements. Agron. J. 112(4): 3195–3215. doi: 10.1002/agj2.20234.
Rog, I., M.G.A. van der Heijden, F. Bender, R. Boussageon, A. Lambach, et al. 2025. Mycorrhizal inoculation success depends on soil health and crop productivity. FEMS Microbiology Letters 372. doi: 10.1093/femsle/fnaf031.
Verbruggen, E., M.G.A. van der Heijden, M.C. Rillig, and E.T. Kiers. 2012. Mycorrhizal fungal establishment in agricultural soils: factors determining inoculation success. New Phytologist 197(4): 1104–1109. doi: 10.1111/j.1469-8137.2012.04348.x.
Wilson, G.W.T., C.W. Rice, M.C. Rillig, A. Springer, and D.C. Hartnett. 2009. Soil aggregation and carbon sequestration are tightly correlated with the abundance of arbuscular mycorrhizal fungi: results from long-term field experiments. Ecology Letters 12(5): 452–461. doi: 10.1111/j.1461-0248.2009.01303.x.
Wu, Q.-S., J.-D. He, A.K. Srivastava, Y.-N. Zou, and K. Kuča. 2019. Mycorrhizas enhance drought tolerance of citrus by altering root fatty acid compositions and their saturation levels. Tree Physiology 39(7): 1149–1158. doi: 10.1093/treephys/tpz039.
Wu, Q.-S., X.-H. He, Y.-N. Zou, C.-Y. Liu, J. Xiao, et al. 2012. Arbuscular mycorrhizas alter root system architecture of Citrus tangerine through regulating metabolism of endogenous polyamines. Plant Growth Regul 68(1): 27–35. doi: 10.1007/s10725-012-9690-6.
Wu, Q.-S., and A. Srivastava. 2017. AMF diversity in citrus rhizosphere. Indian Journal of Agricultural Sciences 87: 653–659.
Wu, Q.-S., and Y.-N. Zou. 2009. Mycorrhizal influence on nutrient uptake of citrus exposed to drought stress. Philippine Agricultural Scientist 92: 33–38.
Xi, M., E. Deyett, N. Ginnan, V.E.T.M. Ashworth, T. Dang, et al. 2022. Arbuscular mycorrhizal fungal composition across US citrus orchards, management strategies, and disease severity spectrum. doi: 10.1101/2022.03.01.482593.
Yuan, M.M., A. Kakouridis, E. Starr, N. Nguyen, S. Shi, et al. 2021. Fungal-bacterial cooccurrence patterns differ between arbuscular mycorrhizal fungi and nonmycorrhizal fungi across soil niches. mBio 12(2): 10.1128/mbio.03509-20. doi: 10.1128/mbio.03509-20.
Zhang, X., G. Dai, T. Ma, N. Liu, H. Hu, et al. 2020. Links between microbial biomass and necromass components in the top- and subsoils of temperate grasslands along an aridity gradient. Geoderma 379: 114623. doi: 10.1016/j.geoderma.2020.114623.
Zhang, L., J. Zhou, T.S. George, E. Limpens, and G. Feng. 2022. Arbuscular mycorrhizal fungi conducting the hyphosphere bacterial orchestra. Trends in Plant Science 27(4): 402–411. doi: 10.1016/j.tplants.2021.10.008.