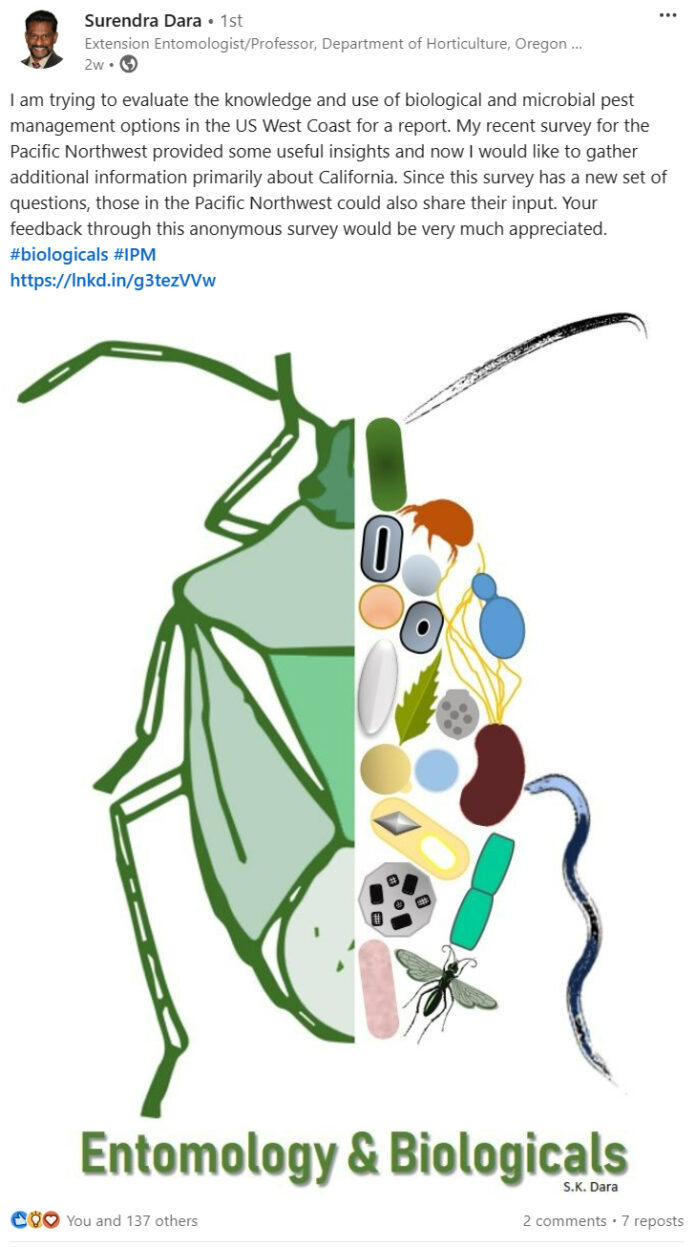
As food production faces the persistent threat of endemic and invasive pests, researchers continue to develop new technologies and strategies for protecting crops from these threats. One such new technology is RNA interference (RNAi) with targeted mechanisms toward specific pests. RNAi can be used as a trait in a crop or as a sprayable product against the target pest. Before delving further into this, here are a few basic details of this biological process that will help understand the RNAi mechanism.
Deoxyribonucleic acid (DNA) in the chromosomes of most living organisms contains genetic code for making proteins that are essential for various biological processes. Ribonucleic acid (RNA) carries the genetic code from DNA to the protein-making factories within the cell known as ribosomes. DNA has two strands of nucleotides (sets of deoxyribose sugar with nitrogenous bases connected by a phosphate group) whereas RNA has only one strand. RNA also differs from DNA in having ribose sugar, instead of deoxyribose, and a different kind of nitrogenous base. The purpose of RNA is to transfer the genetic code from DNA as amino acids are made in ribosomes. A chain of amino acids makes a specific protein. Examples of proteins in insects include juvenile hormones responsible for development and reproductive maturation; ecdysone responsible for molting and metamorphosis; digestive enzymes like amylases, glycosidases, lipases and proteases; and esterases that are important in metabolizing various compounds that regulate behavior, development, insecticidal resistance and other processes.
RNAi involves silencing the expression of a specific gene by double-stranded RNA (dsRNA) pieces (either small interfering RNA or microRNA each containing about 21 to 23 nucleotide pairs) attaching to messenger RNA (mRNA) carrying the code from DNA and thus interfering with the production of a specific protein. RNAi is also known as post-transcriptional gene silencing because the silencing is done after the DNA code is transcribed to mRNA. RNAi is a natural phenomenon that helps organisms to defend against infections or regulate gene expression. For example, when there is a viral infection, cells activate RNAi to destroy virus particles. RNAi-based therapies are currently used in the medical field to treat cancer and neurological issues and to regulate oxalic acid in urine or the low-density lipoprotein cholesterol in blood.
Use in Agricultural Crops
RNAi can be used in agriculture for improving yield or quality, imparting abiotic stress tolerance or pest resistance and incorporating other desirable traits or as biopesticides in crop protection (Bharathi et al. 2023; Chaudhary et al. 2024). Many research studies have been exploring the RNAi potential in agriculture for decades (Fletcher et al. 2020). Modifying plant height in apple (Zhao et al. 2016), rice (Qiao et al. 2007) and tomato (Cheng et al. 2020); imparting drought, salt and heat tolerance in cotton (Abdurakhmonov et al. 2014), abiotic stress tolerance in cereal crops (Dubrovna et al. 2023) and cold tolerance in tomato (Jiao et al. 2024); imparting resistance to blast (Magnaporthe grisea) and leaf blight (Xanthomonas oryzae pv. oryzae) in rice (Jiang et al. 2009), citrus canker (Xanthomonas citri subsp. citri) in citrus (Enrique et al. 2011), late blight (Phytophthora infestans) in potato (Eschen-Lippold et al. 2012), Fusarium head and seedling blight (Fusarium graminearum) in wheat (Cheng et al. 2015), soybean mosaic virus in soybean (Kim et al. 2016); imparting resistance to bollworm (Helicoverpa armigera) in cotton (Mao et al. 2007 and 2011) and resistance to brown planthopper (Nilaparvata lugens) in rice (Zha et al. 2011); and imparting resistance to root-knot nematode (Meloidogyne incognita) in tomato (Dutta et al. 2015) and soybean cyst nematode (Heterodera glycines) in soybean (Guo et al. 2015) are some of the examples of improving crop traits.
The first RNAi crop in the U.S. was corn (SmartStax® PRO) against the western corn rootworm (Diabrotica virgifera virgifera) containing both Bacillus thuringiensis toxins and RNAi technology (Head et al. 2017). With its ability to resist both belowground and aboveground lepidopteran pests, this hybrid is an important IPM tool. This hybrid is also available in Canada for cultivation, and grain and products from the hybrid are approved for consumption in the European Union. RNAi-based crops are not considered genetically modified organisms (GMOs) because they do not contain a foreign gene to express a particular protein like GMOs but use a natural mechanism to silence a particular gene.
In addition to adding desirable traits to crops, RNAi has also been explored or developed for treating plants against pests and diseases. While RNAi crops use the host-induced gene silencing (HIGS) method, RNAi biopesticides use the spray-induced gene silencing (SIGS). SIGS has been explored for controlling Fusarium graminearum in barley (Koch et al. 2016), sucking and/or stem-boring insects in multiple crops (Li et al. 2015; Hunter and Wintermantel, 2021; Jain et al. 2022) and hawthorn spider mite (Amphitetranychus viennensis) in fruit trees and woody ornamentals (Yang et al. 2023). The first sprayable formulation of RNAi-based biopesticide is CalanthaTM from GreenLight Biosciences against the Colorado potato beetle (CPB), Leptinotarsa decemlineata (Rodrigues et al. 2021). The active ingredient is a dsRNA molecule known as Ledprona (Leptinotarsa decemlineata-specific recombinant double-stranded interfering Oligonucleotide GS2). It belongs to a new class of insecticides under group 35 as an RNAi-mediated target suppressor. Applied as a foliar spray, Ledprona suppresses the gene that produces proteasome subunit beta type-5 (PSBT5) in CPB and arrests insect feeding within two to three days after it is ingested, leading to the death of the pest. PSBT5 is an essential protein important in maintaining cellular protein quality by degrading damaged or misfolded proteins or proteins that are no longer needed.
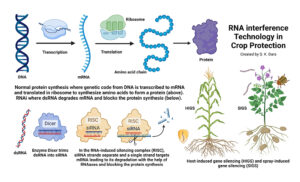
RNAi can also be used to protect honey bees from the Israeli Acute Paralysis Virus (Hunter et al. 2010) and the Varroa mite (Garbian et al. 2012). In field studies, honey bee populations and honey production increased when bees were fed dsRNA for the virus in the presence of virus in the colonies (Hunter et al. 2010). The ectoparasite Varroa mite is a major threat to the honey bee colony health and its management is a significant challenge. When honey bees ingest the mite-specific dsRNA that silences the calcium ion-binding protein known as calmodulin, the dsRNA is transmitted to the Varroa mite feeding on the hemolymph of the bees, resulting in mite mortality (Garbian et al. 2012).
Minimal Environmental Risk
As with any new technology, it is important to consider the impact of RNAi on the environment and non-target organisms. Environmental risks and regulatory aspects of RNAi-based products have been reviewed in various reports (Liu et al. 2021; De Schutter et al. 2022; Christiaens et al. 2022). Microbial activity, UV radiation and other environmental conditions degrade dsRNA, and they are generally less stable in the environment, especially under the field conditions where they are used (Bachman et al. 2020). Studies showed dsRNA degraded within two days in soil and one to three days in aquatic environments (Dubelman et al. 2014; Fishcer et al. 2017). Chen et al. (2023) reported while an RNAi-based biopesticide was highly effective against the 28-spotted ladybeetle (Henosepilachna vigintioctopunctata), a pest of solanaceous crops, it had no non-target effect on the predatory lady beetle Propylea japonica. Similarly, studies showed the dsRNA developed for controlling Varroa mite were safe for honey bees (Tan et al. 2016; Vélez et al. 2016) and the monarch butterfly (Danaus plexxippus) whose calmodulin mRNA has a slight match to the Varroa-active dsRNA (Krishnan et al. 2021).
With regards to Ledprona, the U.S. Environmental Protection Agency (EPA) found it has minimal human and environmental risks due to low application rates, rapid microbial degradation in the environment and physiological barriers and degradation mechanisms in mammals. U.S. EPA also gave Ledprona a “No Effect” determination according to the Endangered Species Act.
Environmental instability is one of the concerns for SIGS, but formulation technology can address this problem. Instead of spraying naked dsRNA, formulating it with layered double hydroxide clay nanoparticles known as BioClay significantly extended the stability of dsRNA. Spraying dsRNA in BioClay provided protection against pepper mild mottle virus and cucumber mosaic virus at least for 20 days, and dsRNA was detected on the leaves 30 days after application (Mitter et al. 2017). Similarly, spraying BioClay-formulated dsRNA 5 days before exposing to virus-containing green peach aphids (Myzus persicae) offered protection against the bean common mosaic virus in cowpea and benth (Nicotiana benthamiana) (Worrall et al. 2019). In a more recent study, BioClay-formulated dsRNA against gray mold (Botrytis cenerea) increased disease protection from one week to three weeks on leaves and five days to 10 days on fruit (Niño-Sánchez et al. 2022).
Arthropod pests and pathogens are resilient and rapidly evolving organisms and can develop resistance to RNAi technology just like they develop to pesticides or transgenic crops. Whether it is HIGS or SIGS, avoiding heavy reliance on one tool and adopting integrated pest management (IPM) and resistance management strategies is crucial even when using RNAi. An IPM strategy that takes advantage of multiple tools will minimize the risk of resistance development while achieving desired pest suppression.
Resources
Videos about RNAi: https://youtu.be/xDg6pu7HWz4 and https://youtu.be/cK-OGB1_ELE
For the full list of references, visit ucanr.edu/blogs/blogcore/postdetail.cfm?postnum=59299.